Ever since the telescope was first used by Galileo to study the heavens astronomers have built bigger and bigger telescopes to aid them in their work. To an astronomer the bigger the telescope the better for two basic reasons, the first reason is simply that the bigger the telescope the more light it can collect. This extra light allows objects that are too dim to be seen with the unaided eye to become visible. Point even a small telescope at a portion of the sky where you see only one or two stars and suddenly you’ll see dozens of stars because the telescope has a larger area to gather more light from those dimmer stars.
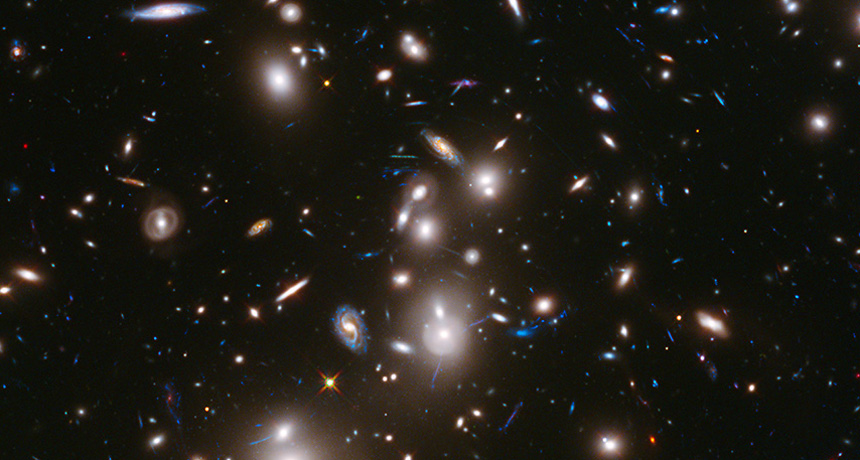
The second reason is that a telescope, because of its ability to magnify what it sees, can separate two objects that appear to the naked eye to be a single object. Soon after a child receives their first telescope, and after a few nights looking at the Moon and a few planets they will turn to look at a star like Rigel or Spica in order to see how a single star becomes two or even more stars in their new ‘scope.
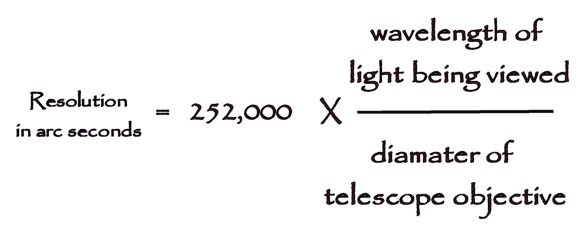
This ability to resolve the details of distant objects is a function of the size of the telescope divided by the size of the wavelength of the Electromagnetic waves it is designed to collect. For an optical telescope the wavelength of visible light is very small so they tend to have a lot of resolution. For radio telescopes however the waves they collect can have wavelengths that are centimeters or even meters long. In fact at a low frequency like 3 Mega-Hertz (MHz) the wavelength is actually a full 100m.
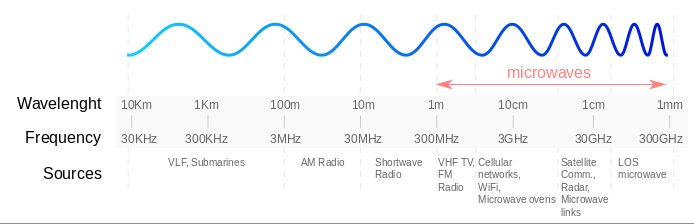
This has always made the ‘images’ produced by radio telescopes much ‘fuzzier’ than those from optical telescopes. Over the last couple of decades however radio astronomers have developed a workaround thanks to the enormous progress in computer and communications technology. What they have done is link as many as a dozen radio telescopes in different parts of the world together electronically so that the signals they collect are added together by a supercomputer, effectively making the separate telescopes into a single one with a size of nearly the entire planet.
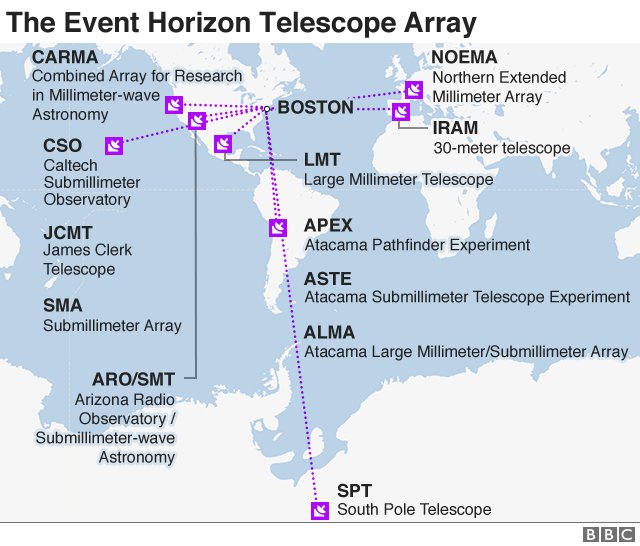
As mentioned in a previous post 17 April 2019, this technique is being used to provide the most detailed images ever obtained of the supermassive black holes at the center of galaxies along with other objects of interest. The telescopes used in these projects however were not originally designed to be used in conjunction with other scopes.
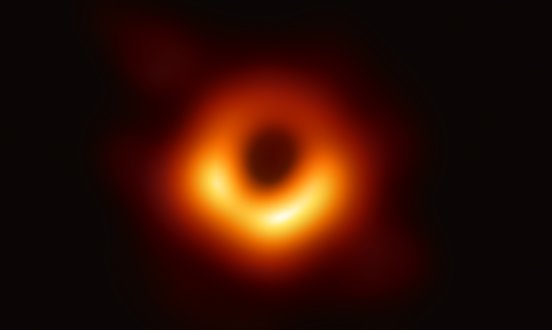
Now a large array of radio receivers that are designed to act as a single radio telescope has been constructed at sites spread out over a sizeable portion of the Earth’s surface. Known as the LOw Frequency ARray or LOFAR project and originally funded as a national program by the government of the Netherlands LOFAR has now grown to include most of the countries of Western Europe.
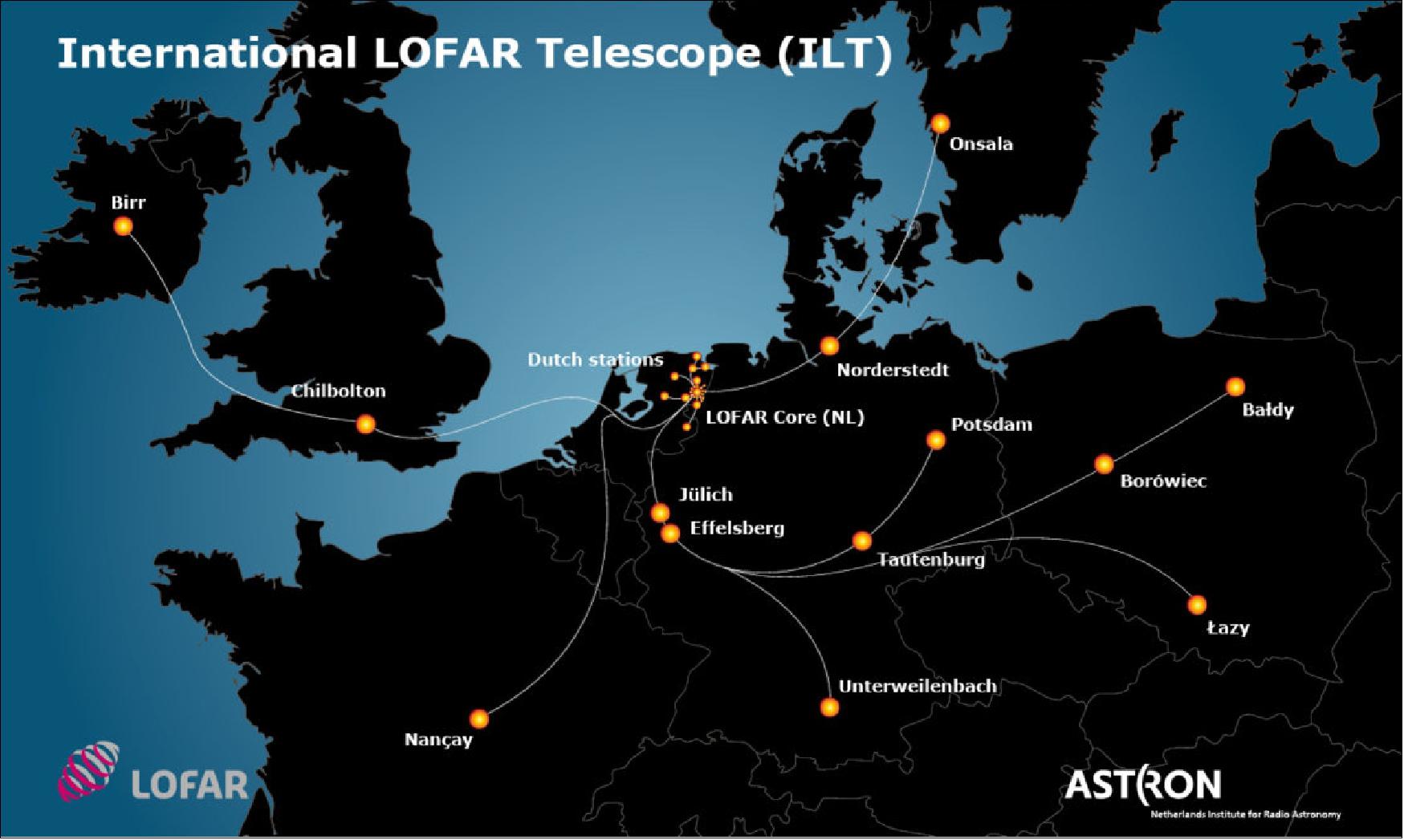
Currently LOFAR consists of 38 stations in the Netherlands, 24 core stations in the province of Exloo and 14 remote station spread around the rest of the country. There are also 14 international stations, 6 in Germany, 3 in Poland and one each in Ireland, the UK, France, Sweden and Latvia with a fifteenth station under construction in Italy. Each station is composed of 96 Low Band Antennas (LBAs) that receive signals between 10 and 90 MHz, along with 48 High Band Antennas (HBAs) that receive in the 120 to 240 MHz band. All together this makes for a total of more than 70,000 antennas in the LOFAR array. Each station also has the computer facilities to completely digitize all of the signals received by its antennas so that the data can be combined with those from all the other stations making LOFAR the highest resolution radio telescope ever built.

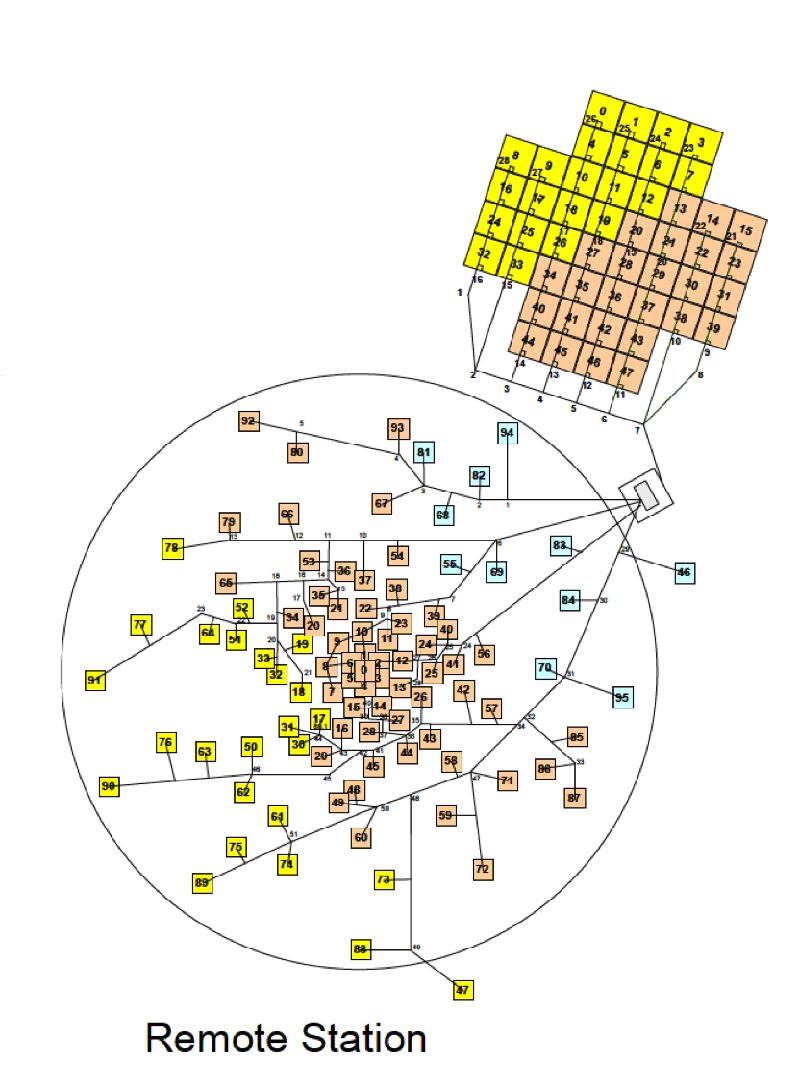
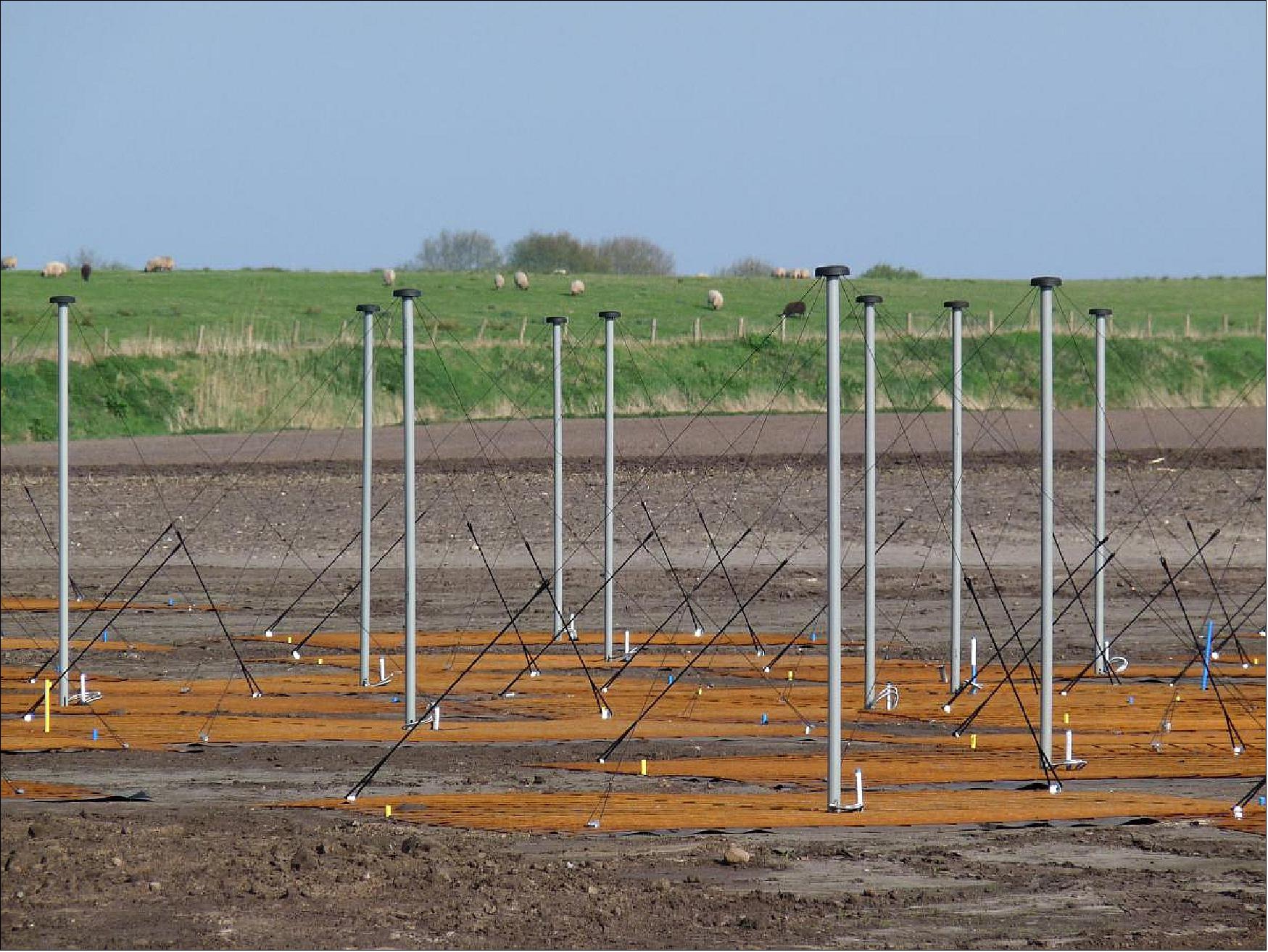
The initial results published by the LOFAR consortium have dealt with detailed studied of the radio emissions from the supermassive black holes at the center of galaxies. When combined with optical images of the galaxy containing the black holes, see image below, astronomers can get a much more complete picture of the influence of supermassive black holes on their host galaxies.
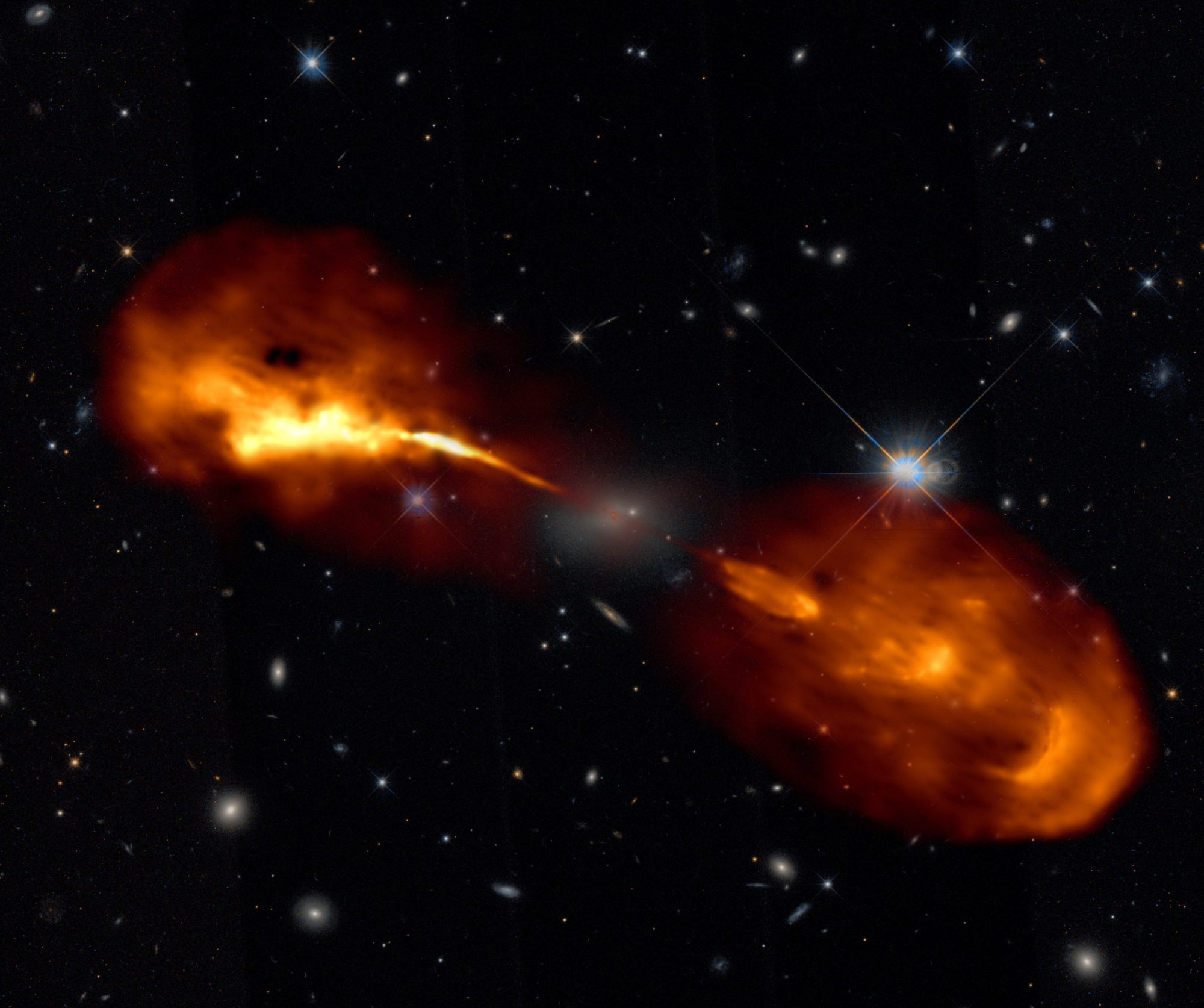
Other studies planned for LOFAR include a full scale investigation into the early period of the Universe known as the ‘Period of Re-Ionization’ when the first stars and galaxies heated the gas and dust created by the big bang. LOFAR will also be employed to study transient sources like pulsars and fast radio bursts (FRBs). Closer to home LOFAR will study our Sun and in particular the solar wind of high energy particles emitted by the Sun.
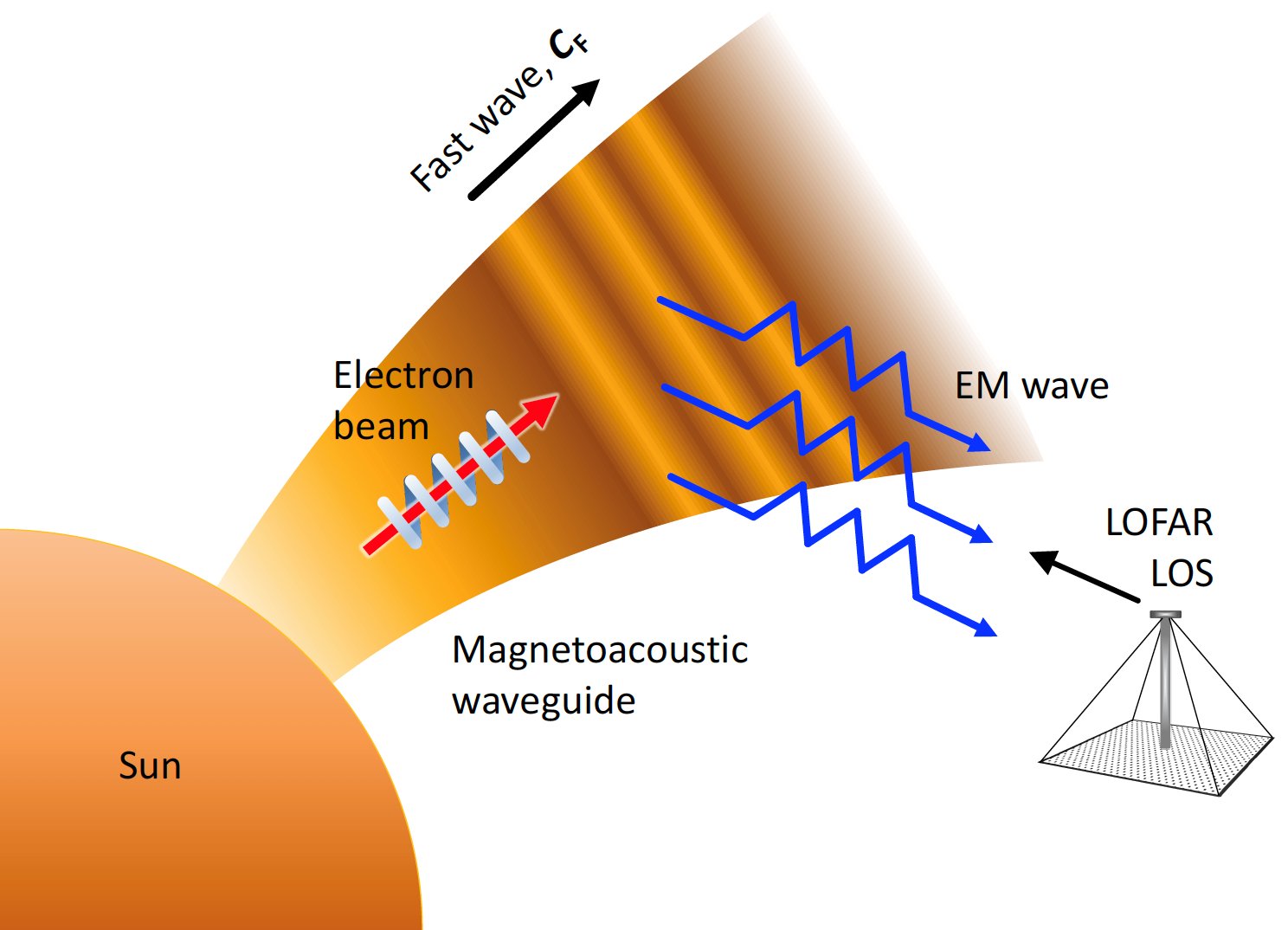
Best of all the LOFAR partnership has already submitted its plan to the EU for LOFAR2.0, a major upgrade to the system that is planned to commence in 2022. One can only guess at what wonders that system will reveal.