With all of the mass shootings taking place across the United States nowadays it seems that we’ve all just come to accept the massive amounts of bloodshed as ‘normal human behavior’ against which nothing can be done. In keeping with this philosophy the National Rifle Association (NRA) along with their Republican lawmakers continue to assert the premise that ‘The only thing that can stop a Bad man with a Gun is a Good Man with a Gun.’

But a premise is not an observed fact, and to be considered true a it must stand the test of comparison against actual observations. That means that the premise about good men stopping bad has to be judged by those shooting incidents where the bad man with a gun was stopped by one or more good men with guns. I would like to do just that using the recent mass shootings at Robb elementary school in Uvalde Texas and the Greenwood Park Mall in Greenwood, Indiana. I will argue that these two events represent the two extremes in the reactions of the ‘good man’ to the problem of the ‘bad man’. As such they are what a physicist would call boundary conditions which can be used to give insight into all of the possible outcomes of the ‘good man / bad man’ premise.
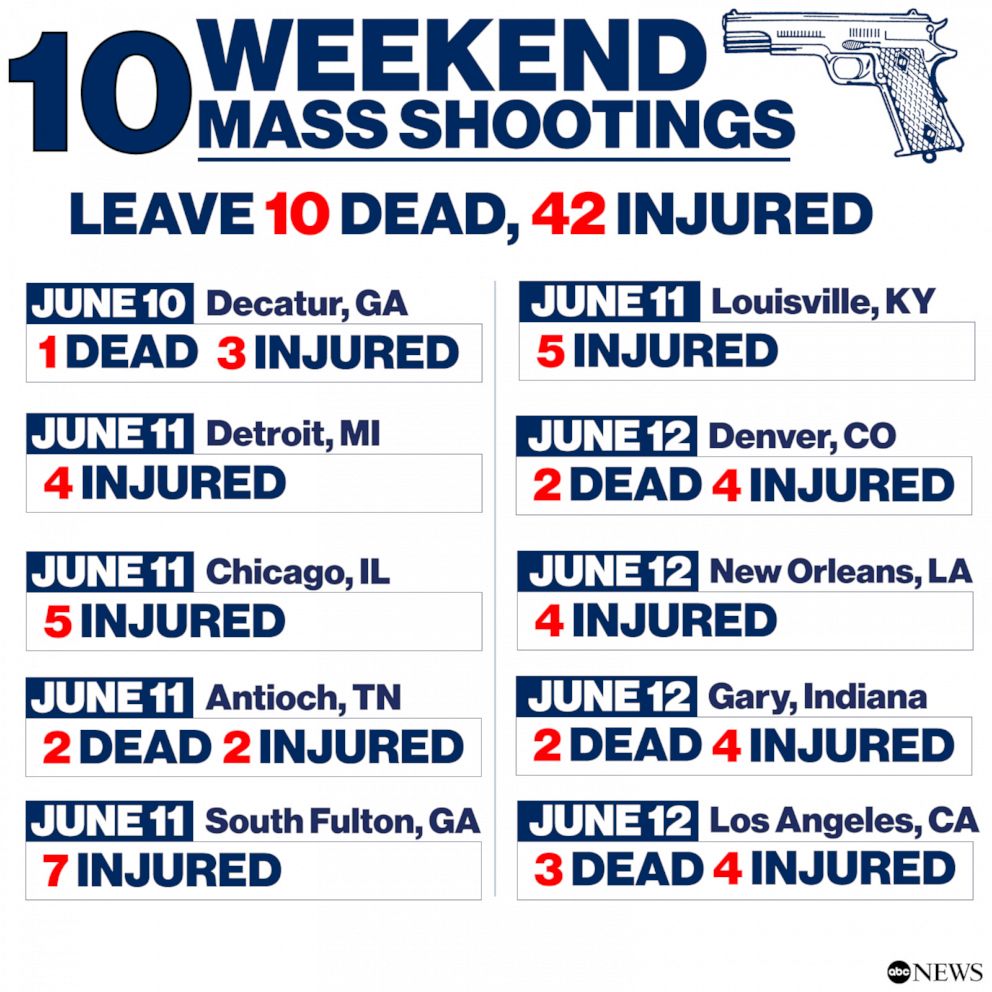
In both of these incidents the shooter acted without any immediate provocation or intent to achieve some rational goal. Instead the perpetrators simply carried an arsenal of weapons to a place where a large number of innocent people would be and begin firing with the aim of killing as many people as possible. In neither incident did the assassin have a prior record of violence sufficient enough to prevent them from purchasing their weapons legally, nor for each killer has a motive has been discovered for their heinous acts. For these reasons the shooter in both the Uvalde, Texas and the Greenwood, Indiana mass shootings certainly can be classified as a ‘Bad Man with a Gun’.
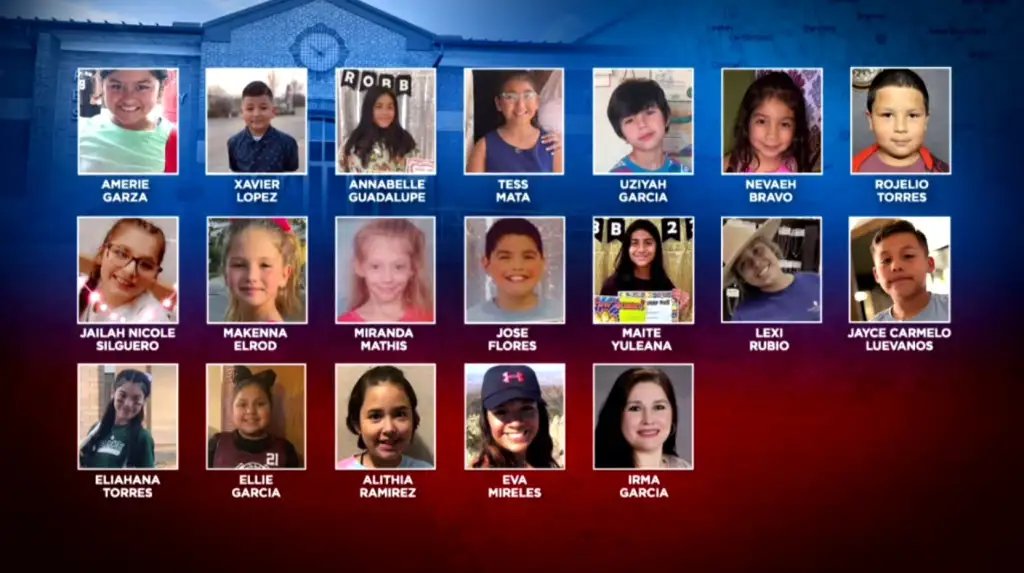
Also, in both Uvalde and Greenwood the bad man with a gun was demonstrably stopped, killed actually by a good man with a gun. In Uvalde the shooter, whom I will not name, was killed when local police stormed the schoolroom where he had barricaded himself. In the Greenwood shooting it was an ordinary citizen, just someone who was himself licensed to carry a firearm, who engaged and killed the mass shooter. The main difference between the two confrontations is in the competence of, and most importantly the speed with which the good man reacted to the life and death situation at hand.
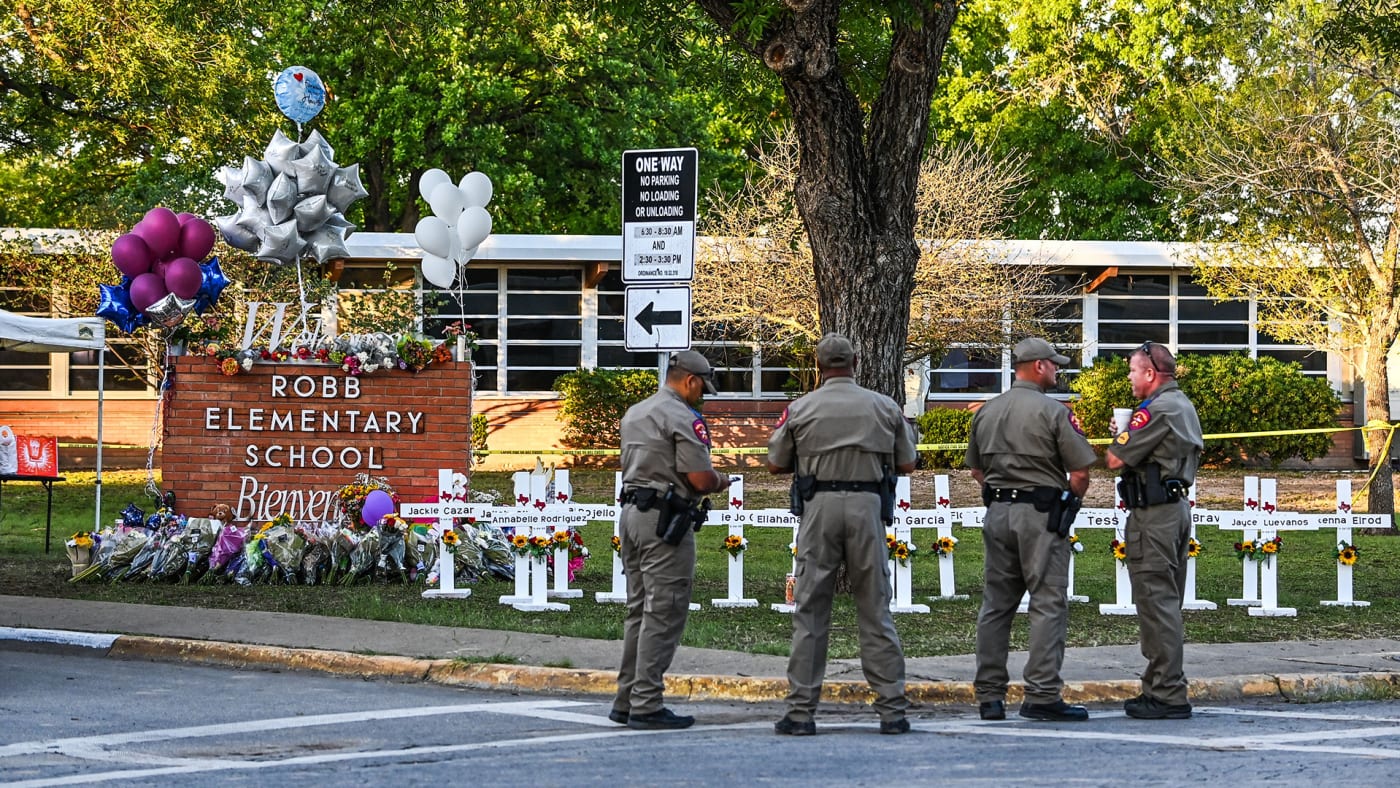
At Uvalde a laundry list of ‘systemic failures’ along with an unwillingness to act by senior law enforcement officers led to a 77 minute delay, after the shooter had begun his rampage, before the assassin was finally confronted and subdued. During all that time 19 children and two of their teachers lay dead or dying while others who still lived were at the mercy of the shooter. Certainly part of the reason for the delay was the large number of different law enforcement agencies that responded to the active shooter alert. Of the 376 good guys who showed up at Robb Elementary 149 were members of the U.S. Border Patrol while 91 were state police, the vaulted Texas Rangers. It seems as though these state and federal officers thought that local police should take charge because of their superior knowledge of Robb Elementary and the people there.

And there were more than enough local police at the scene. 12 Arredondo’s Sheriff’s Deputies along with 25 members of Uvalde’s police force of whom 5 were school police. All in all there were simply too many cops from too many agencies with no one willing to step up and take charge. Confusion as to room keys and whether any of the kids in the room could still be alive added to the chaos but in any case Robb Elementary School in Texas is an excellent example of just how badly a ‘good man with a gun’ can handle a ‘bad man with a gun’. In other words, it is undeniable that in some cases good men cannot be relied upon, some better solution must exist and must be found.

The shooting at Greenwood Mall in Greenwood, Indiana is just the opposite. When a shooter opened fire at the Mall’s Food Court with his assault rifle local citizen Elisjsha Dicken was having lunch with his girlfriend. As he heard the gunshots Elisjsha, who is legally permitted to carry a firearm in the state of Indiana, immediately took out his own pistol and calmly fired ten rounds, mortally wounding the shooter. Unfortunately, despite Dicken’s heroic actions the killer still managed to kill three innocent people and wound a fourth before being taken him down.
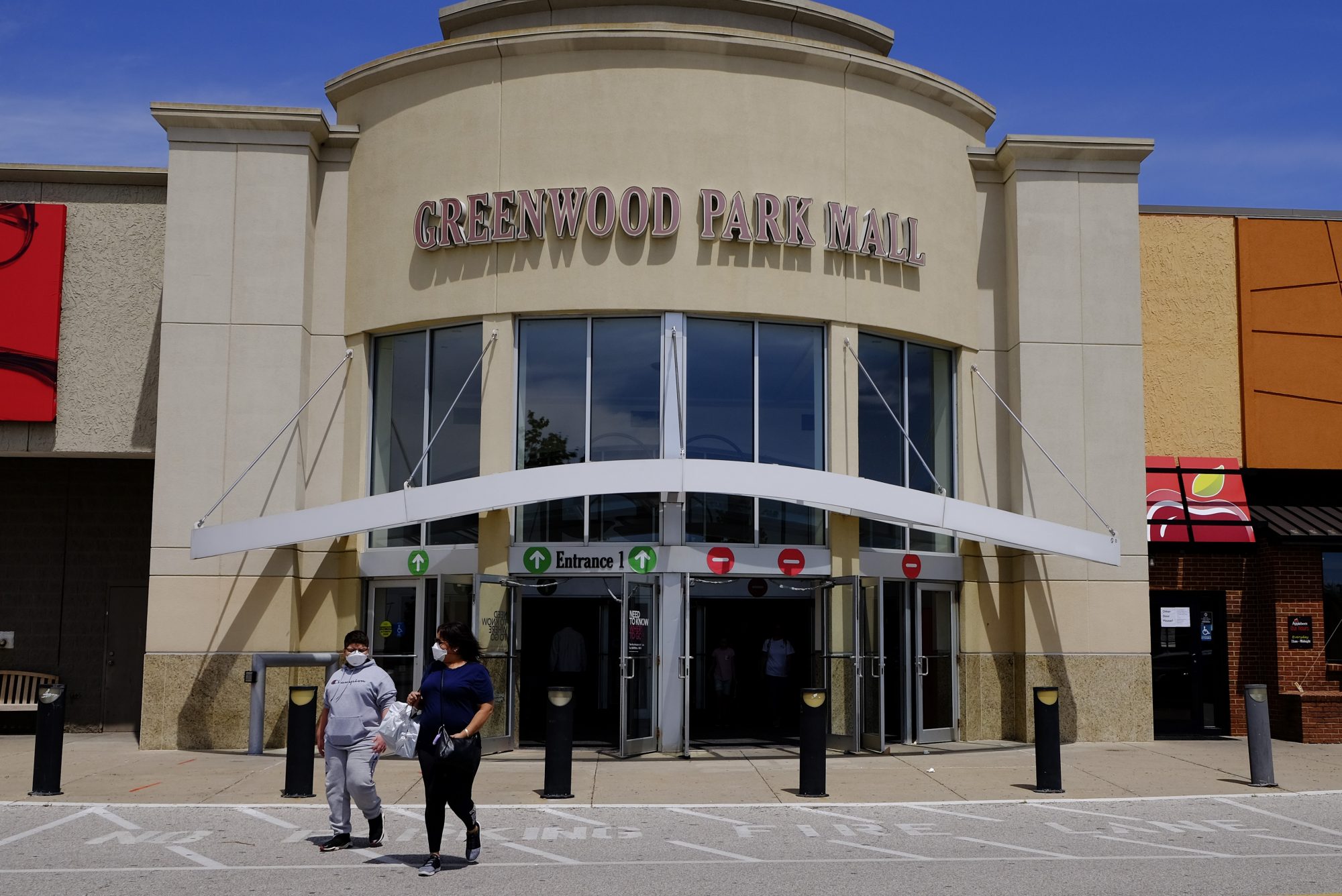
At Greenwood the good man reacted immediately, stopping the assassin before he could harm anyone else, therefore it can be argued that Elisjsha Dicken represents the best case scenario of the ‘Good Man with a Gun stopping a Bad Man with a Gun’. Three innocent people still got killed however, the best case scenario was still a mass shooting with multiple dead and wounded.
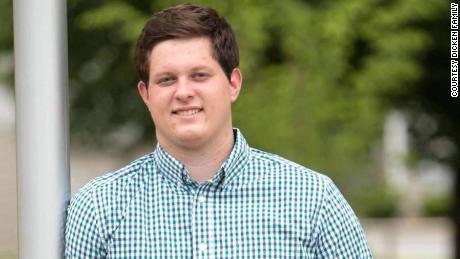
I have argued above that Uvalde and Greenwood can be considered as approximating the worst and best case of the possible outcomes for the ‘The only thing that can stop a Bad man with a Gun is a Good Man with a Gun’ premise. If that is so it is clear that even the best case scenario is still horrific and every other possibility progressively worse making the premise demonstrably false.
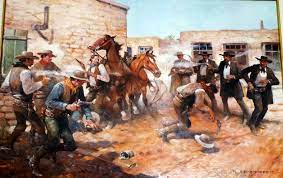
Therefore, if we do in fact want to try to reduce the level of gun violence in this country, and yes it is true we cannot even hope to completely stop it, then we must find a new premise to test. Of course everyone already knows what that premise is, ‘The best way to stop a Bad man with a Gun is to NOT LET THEM GET A GUN in the first place’.
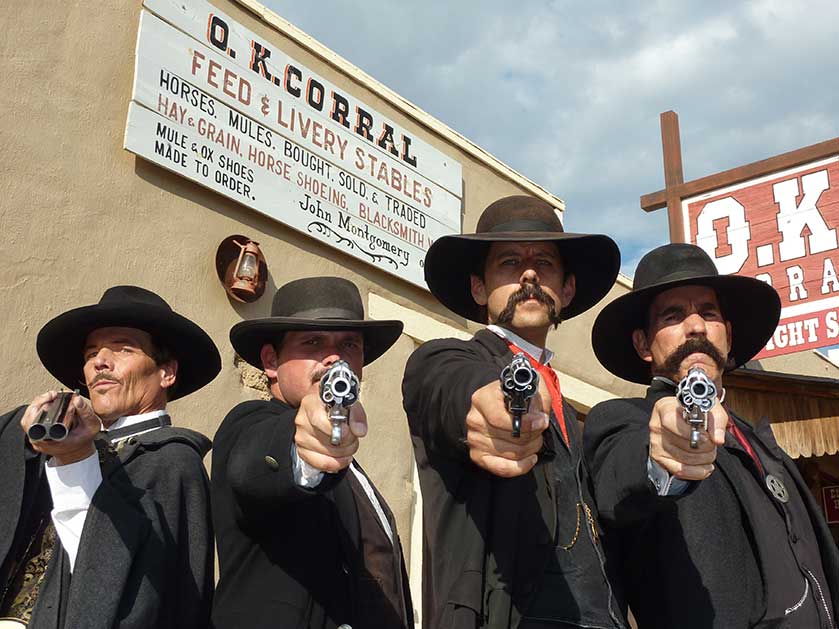
In other words gun control, eliminating military style weapons completely along with high capacity magazines. At the same time we must adopt stricter background checks to keep people with mental problems from acquiring firearms. None of these suggestions have to adversely effect legitimate hunters or those who want to purchase a gun to protect their homes. They will however reduce the current high frequency of murders in this country, not just the mass shootings. Gun violence in the US has grown tremendously over the last thirty years, it’s time to finally do what we all know is the only thing that will actually work.