Ever since physicists began experimenting with the nuclei of atoms they realized that producing energy by the fusion of light nuclei had several advantages over the splitting, fission of heavy nuclei. For one thing fusion simply produces more energy than fission but more importantly fusion does not produce the large amounts of radioactive waste that are the biggest problem with our current nuclear power plants.

The big problem with using nuclear fusion to generate power is that it’s so hard to initiate. Whereas a nucleus of Uranium 235 is so unstable that almost any disturbance, like a stray neutron striking it, will cause it to split into smaller nuclei a hydrogen nuclei, really just a proton, will electrostatically repel any other proton making it very difficult to force them to fuse together. This is because the strong nuclear force keeping nuclei together is incredibly short ranged; you practically have to get the protons to touch in order for them to stick. The electromagnetic (EM) force on the other hand obeys an inverse square law like gravity does and never really goes to zero.
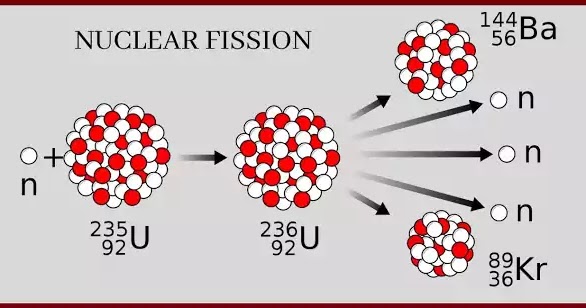
In the center of the Sun fusion is only possible because of the extreme pressures and temperatures there. So in order to generate fusion in the labouratory scientists and engineers have for over 60 years tried to replicate the conditions at the center of the Sun.
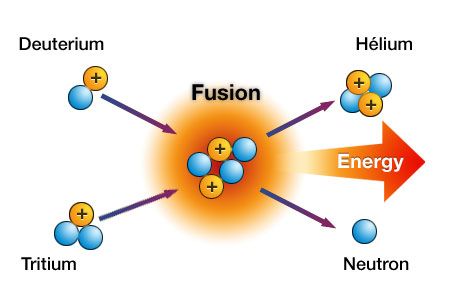
The usual design for these attempts has been a doughnut shaped vacuum chamber inside a very powerful magnetic field known as a Tokamak. Inside the Tokamak an ionized gas known as a plasma is heated to millions of degrees Celsius and contained by the magnetic field. Once the fuel reaches a high enough temperature some of the nuclei begin to fuse releasing energy that can then be converted into electric power. The ITER fusion reactor, which is currently under construction in France, is expected to operate at a plasma temperature of 150 million degrees Celsius!
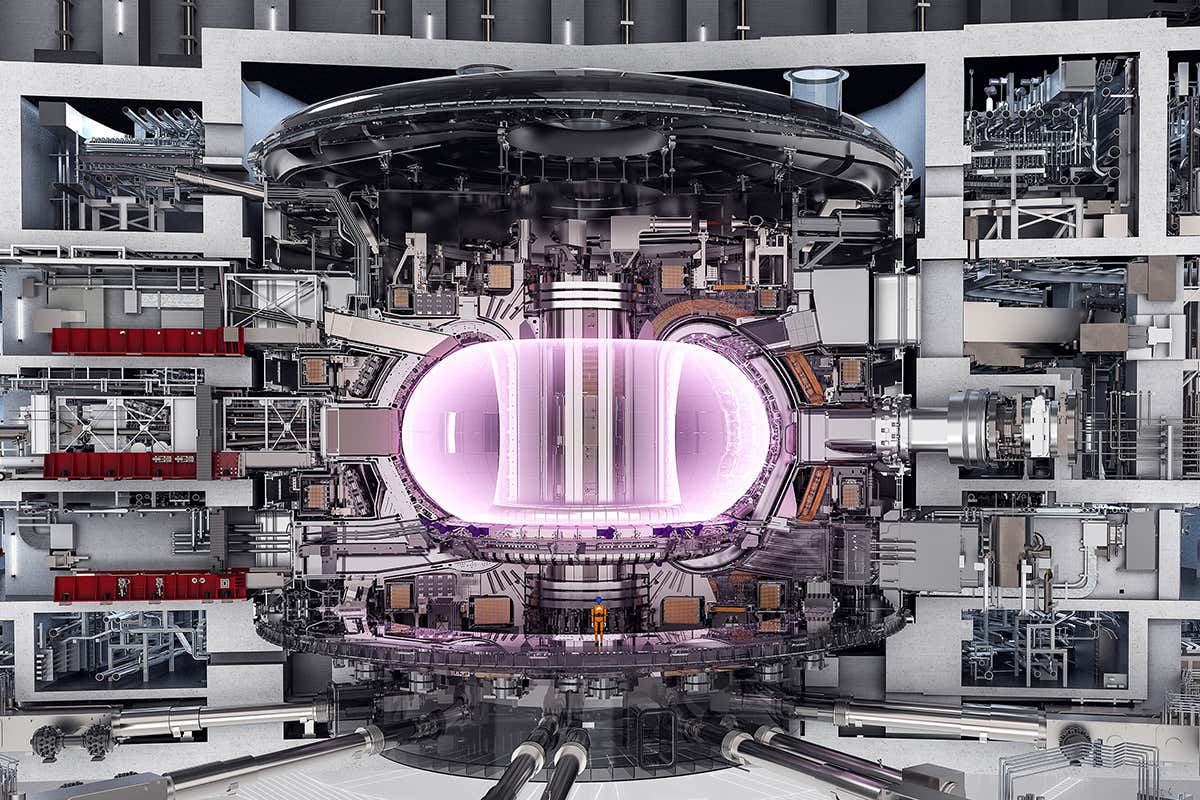
The fuel used in most fusion experiments are heavy isotopes of hydrogen, also known as deuterium and tritium, D-T, which consist of a single proton attached to either a single neutron or two neutrons respectively. The reason that heavy hydrogen is the fuel of choice is that with only a single proton in each nuclei the repelling force is minimized, the greater the number of protons the greater the repelling EM force and the higher temperature needed to force fusion to occur.
D-T has one big drawback however, about 80% of the energy released is by the emission of free neutrons. These neutrons are not only dangerous to living tissue, and therefore require a large amount of shielding around a fusion reactor, but it is also difficult to covert the kinetic energy of neutral particles into useful electric power. High energy charged particles however can be directly converted in electric power by several techniques, moving electric charges is the definition of electric current after all.

For this reason there has been a lot of interest recently in several other fusion reactions that are considered to be ‘aneutronic’ with only a very small amount of the energy generated being released by neutrons, the majority coming from charged particles. Three aneutronic reactions in particular are being studied:
Helium Three, that’s two protons with a neutron, with Deuterium
He3+D→He4+p +18.3 MeV of Energy
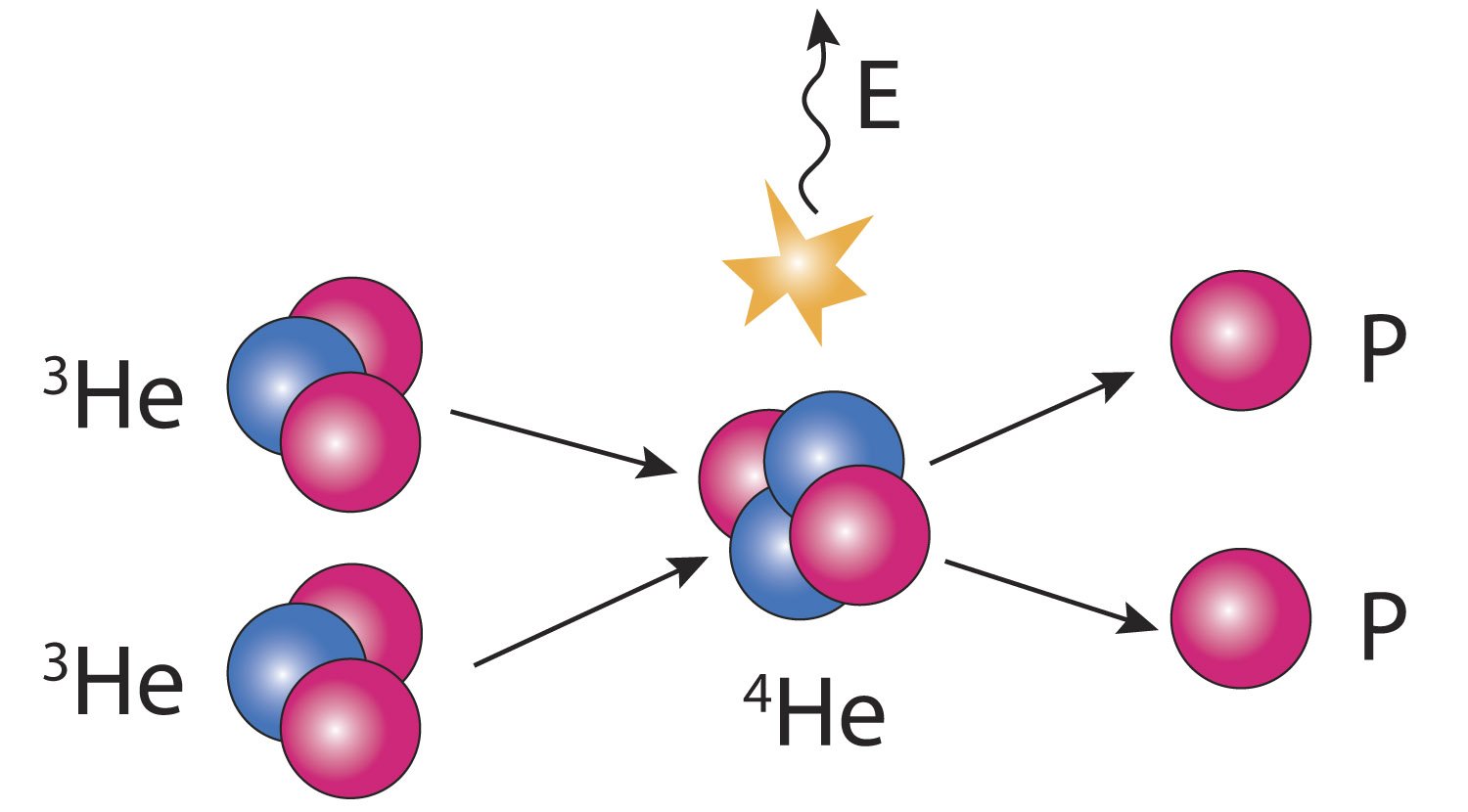
Lithium 7, three protons and four neutrons, with a proton
Li7+p→2He4 + 17.2 MeV
Boron 11, five protons and six neutrons, with a proton
B11+p→3He4 + 8.7 MeV

The first reaction, Helium 3 and Deuterium requires the lowest temperature of any of the aneutronic reactions and releases the most energy of any of the three reactions. The problem is that Helium 3 is virtually non-existent here on Earth. Traces of Helium 3 have been found in samples of rocks brought back from the Moon and which space scientists theorize was produced by billions of years of the solar wind striking the Lunar surface.
Science fiction writer have picked up on this availability ofthis potential energy source on the Moon and several novels have been written using Helium 3 mining as a reason for establishing a Lunar colony. However obtaining even a few useful kilograms of Helium would require processing millions of kilos of Lunar regolith, a endeavour well beyond our current space technology. Maybe one day a large Lunar colony will supply Earth with fuel for its fusion reactors, but that’s many decades in the future.
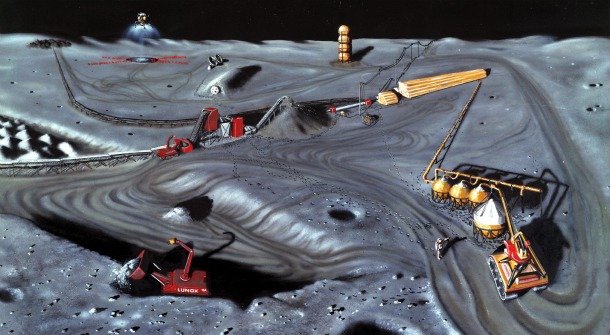
That leaves Lithium and Boron as possible fuels for an aneutronic fusion reactor and while neither element is especially abundant there is still more than enough to keep thousands of fusion reactors running for centuries. As shown in the reactions above Lithium fusion releases the greater amount of energy but is generally not considered aneutronic because as much as 10% of the energy is released as neutrons by the secondary reaction:
Li7+p→Be7+n
Boron on the other hand releases less than 1% of its energy by neutrons but also releases less than half the energy of the other two. Both lithium and Boron would require a plasma temperature of more than 600 million degrees Celsius in order to produce a sustained fusion reaction.

So there you have it. Aneutronic fusion may provide some definite advantages over heavy hydrogen fusion but because of the much higher temperatures required it’s difficult to see how they can be implemented any time in the near future. Without some unexpected technical breakthrough it seems inevitable that the first nuclear fusion reactors will be based on heavy hydrogen as a fuel.
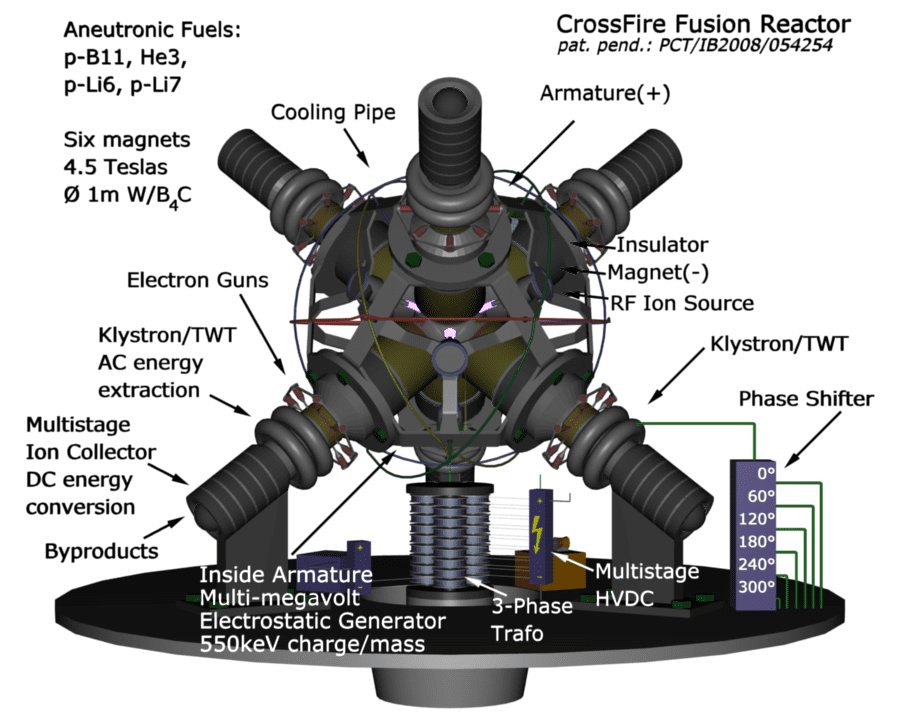
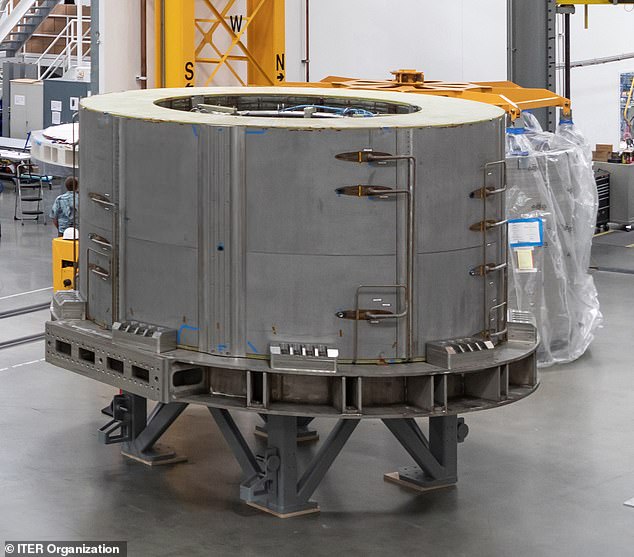
Which is why it’s a sign of progress that the most powerful magnet ever built has been shipped from its manufacturer General Atomics in the United States to southern France. The magnet is the first of six sections of what will become the central core of the ITER reactor. At a 18m in height and 4m in width with a mass of 100 metric tonnes the section cannot be transported by road and is being shipped by a specially prepared ship to the ITER site. Once all six sections are assembled the magnet will produce a field of 13 Tesla or about 280,000 times the strength of the Earth’s magnetic field. It is hoped that the first section will be installed by November.