Two of the greatest mysteries in all of science concern the nature of Dark Matter and Dark Energy which combined are estimated to make up as much as 95% of the matter and energy in the Universe. Dark Matter was first proposed in the 1920s to explain the rotation curves of the galaxies, which when all of the visible matter is taken into account, do not appear to obey Newton’s law of gravity. Dark Matter, particles that only interact with other particles by their gravitational attraction, is supposed to account for this discrepancy. The particles predicted by theories of Dark Matter have been given the generic title of WIMPs, which stands for Weakly Interacting Massive Particles. Trouble is that no particles of a type that could be called Dark Matter have ever been discovered.


Dark Energy on the other hand, was proposed in 1998 to account for the shocking discovery that the Universe was not only expanding, it was accelerating its expansion. Something was pushing the galaxies apart and for want of a better name it was called Dark Energy, although many physicists would rather refer to it as Dark Pressure. We know almost nothing else about it.
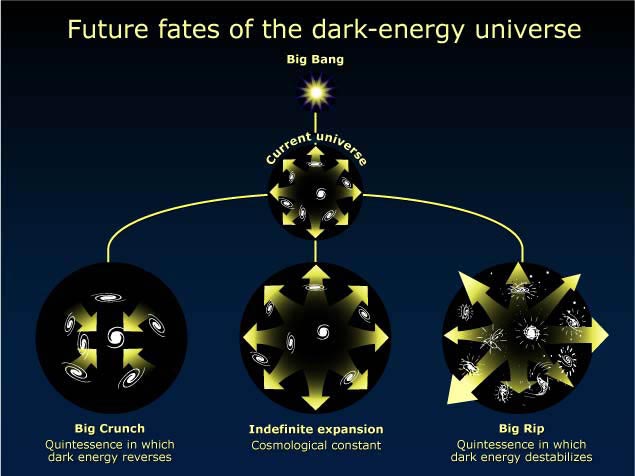
Since Dark Matter is supposed to be made up of WIMPs, particles something like those we’ve been studying for decades experimental physicists have put a good deal more effort into trying to detect them than Dark Energy, which as I said we know almost nothing about. One of the current experiments designed to detect WIMPs is the XENON series of detectors operating at the Gran Sasso National Labouratory in Italy. The Gran Sasso facility is one of number of High Energy Physics labouratories that have been constructed deep underground, often in old mines, in order to help shield them from the cosmic rays that rain down from outer space.

To date there have been three XENON detectors with a fourth just beginning operation, all are designed to provide two distinct signals whenever a WIMP ‘bumps’ against an atom of liquid Xenon. The liquid Xenon is contained within a ‘time projection chamber’, see image below. The liquid Xenon is kept at its boiling point, 165 Kelvin or -108 degrees Celsius, and there is a layer of gaseous Xenon at the top of the chamber. At both the top and bottom of the chamber are arrays of photomultiplier tubes (PMTs). There is also a high voltage electric field across the chamber, positive at the top, negative at the bottom.

When, and if a WIMP strikes an atom of liquid Xenon it knocks off an electron causing a spark of ultraviolet light at a wavelength of 178 nm in a process known as scintillation. This signal is picked up by the lower PMTs and is referred to as S1.
The electron then begins to drift upward at a known speed, the deeper the collision with the WIMP took place the longer it takes for the electron to reach the top. Once the electron reaches the top of the liquid layer it pops out into the gaseous Xenon where it accelerates rapidly knocking into atoms and ionizing them. This ionization releases more photons of light that are picked up by the upper PMTs as signal S2. The X-Y location of the original collision is determined by the location of the PMTs that picked up S2. The Z coordinate, the depth in the liquid Xenon where the collision occurred is calculated from the time difference between S1 and S2.

The first detector to be installed at Gran Sasso contained 15kg of liquid xenon as was known as XENON10. This experiment was operated between October of 2006 and February of 2007 as a proof of concept model and an analysis of the data provided by 58 days of operation found no excess signals that could have been caused by a WIMP collision.
The second experiment, called XENON100, contained 165 kg of liquid xenon and was operated between 2008 and 2014. Over several data collecting runs the detector found no excess signals beyond that which would be expected by random background events.
The latest experiment to complete its data collection run is known as the XENON1T and contains 3.2 tonnes of ultra-pure liquid xenon. This detector began operation in 2014 and gathered 278.8 days of data before being taken off line in order to make space for its successor. During its time in operation the XENON1T experiment recorded 285 events, 53 more events than were theoretically expected by random collisions with radioactive particles. These 53 signals are the first evidence for possible Dark Matter particles, specifically a type of theoretical particle known as an axion.

But a new theory suggests that the 53 events could be the sign of something even more exotic, particles of Dark Energy. In a paper written by astrophysicists at Cambridge University’s Kavli Institute for Cosmology and published in the journal Physical Review D the signals detected by XENON1T could have been caused by a hypothetical particle that screens the short range effects of Dark Energy and which the researchers have christened ‘Chameleons’. The idea is that; while Dark Energy is dominant over a distance scale of the entire Universe, at smaller scales, say within our galaxy, it is almost imperceptible, so some form of screening is necessary. The Chameleon particles would provide that screening.

According to Doctor Sunny Vagnozzi, first author on the paper, “Our chameleon screening shuts down the production of Dark Energy particles in very dense objects…It also allows us to decouple what happens in the local, very dense Universe from what happens on the largest scales, where the density is extremely low.”
This whole idea of screening Dark Energy on the local scale while allowing it to have full effect at the cosmic scale is very new and so far without any observational data to back it up. So the question of just what are those 53 excess events detected by XENON1T, Dark Matter, Dark Energy or just some contamination inside the containment vessel will have to wait.
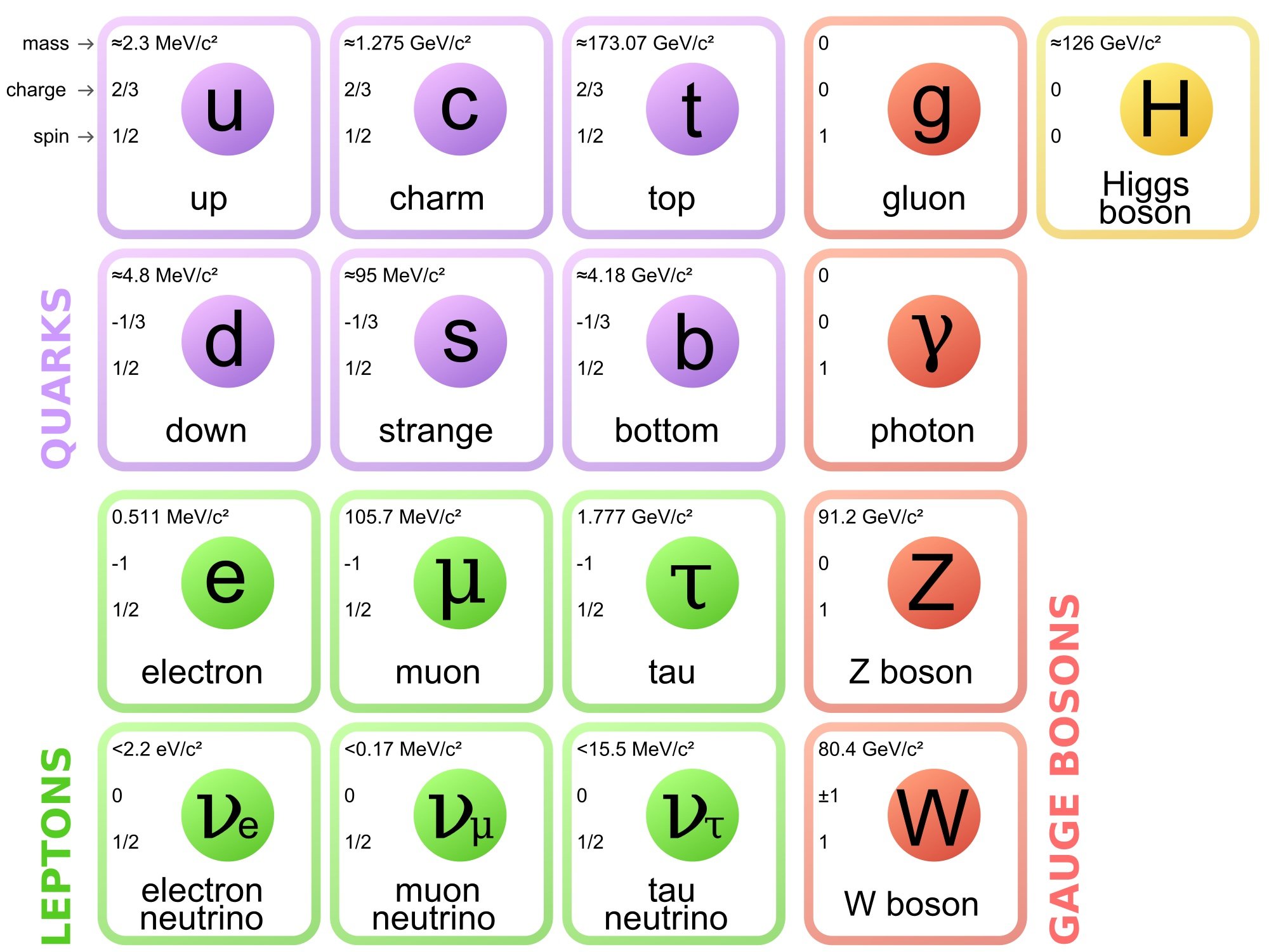

The XENONnT experiment, with 8 tonnes of Xenon gas, came on line just a year ago and is collecting data even now. Perhaps we’ll have the answer in a very few years.