I took in a physics seminar at my old alma mater Drexel University on November 30th. I like to stop down once in a while to see what’s changed, a lot, as well as see who’s there that I still remember, seems like fewer each time.
The topic of the seminar was certainly one that interested me, Neutrinos; a kind of sub-atomic particle so difficult to detect it has been called a ghost particle. The German physicist Wolfgang Pauli first predicted the existence of neutrinos as a way of making the books balance in the radioactive process called beta (β) decay. Careful studies of the process showed that some energy was missing, and the angular momentum before and after didn’t match. Pauli suggested that if another particle was involved, one without electric charge and little or no rest mass, it could account for the differences while being very difficult to detect. The images below show the Nobel Laureate along with a diagram of the β decay process.
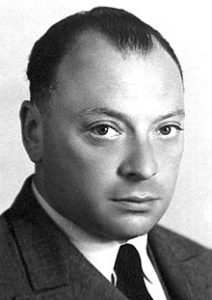
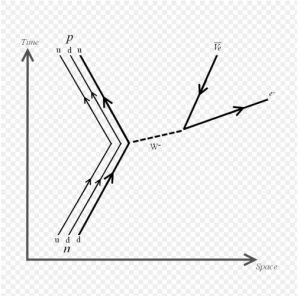
So difficult were neutrinos to detect that it took more than twenty years to prove that they existed. In fact neutrinos react with normal matter so rarely that while about ten billion (billion with a b) neutrinos are flying through your body every second only two or three will interact with a particle inside you during your entire life. Even today the way we study neutrinos is to arrange for zillions to fly through a detector so sensitive it can measure the properties of the one or two that interact.
Arranging that intense beam of neutrinos, and building that detector is the job of Doctor Sowjanya Gollapinni of the University of Tennessee at Knoxville. Dr. Gollapinni is one of the chief researchers of the MicroBooNE experiment currently running at Fermilab outside of Chicago along with being one of the chief designers of the future Deep Underground Neutrino Experiment (DUNE).
The MicroBooNE, BooNE stands for Boosting Neutrino Experiment by the way, is a new type of detector using a design known as a Liquid Argon Time Projection Chamber (LArTPC). In the detector scattering events (really just two particles bouncing off of each other) between neutrinos and Argon atoms occur inside a very large, uniform electric field. The electric field pulls the ionized atoms generated by the collision toward an incredibly fine mesh of detecting wires. The resulting data plots are then interpreted to determine the kind of neutrino (see below) as well as its energy. The images below show the first high-energy neutrino collision captured by MicroBooNE along with the first recorded cosmic neutrino event.
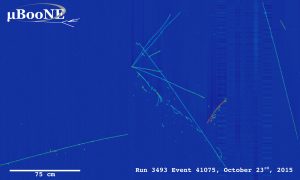
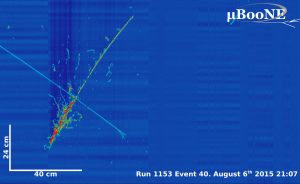
One of the reasons I like MicroBooNE so much is that it uses the Fermilab Tevatron as its source of high-energy neutrinos. The Tevatron was the world’s most powerful ‘atom smasher’ until the Large Hadron Collider (LHC) at CERN took the top spot in 2008. In the world of particle physics however being number two gets you nothing so the physicists at Fermilab have been working hard to reconfigure their equipment in order to continue to study new physics and MicroBooNE is a big part of that effort.
After talking about some of the results from MicroBooNE Dr. Gollapinni spent a little time talking about the next generation neutrino detector known as the Deep Underground Neutrino Experiment or DUNE. As shown in the figure below, DUNE will have two detectors, one just a short distance from the neutrino source at Fermilab while the second will be buried deep inside the Homestake Mine in South Dakota, a distance of 1300 kilometers away. When completed the DUNE detectors will be 400 times larger then MicroBooNE providing 400 time the data.
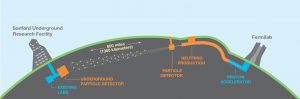
Now the reason for having a second detector a long distance away is to give the neutrinos produced at Fermilab time in order to change from one type or flavour of neutrino to another. You see one of the things we do know about neutrinos is that there are three flavours. One flavour is associated with the familiar electron, a second is associated with a particle called the muon who is like a heavy cousin of the electron while the third is associated with an even fatter cousin called the Tau particle. Even stranger is the fact that the three flavours will oscillate from one kind to another. Learning more about this oscillation process is one of the major goals of DUNE.
At the end of her discussion Dr. Gollapinni mentioned some preliminary but very exciting news. The results so far from MicroBooNE and several other neutrino experiments indicates, just indicates right now, the possible existence of a fourth flavour of neutrino, which would be a stunning result if proving to be true. Right now it’s just an indication, hopefully the DUNE experiment, scheduled to start collecting data in 2024, will give us the answer.
During the question period one of the students who were attending asked Dr. Gollapinni how many flavours of neutrino she thought there were and she answered ‘Well if we find a fourth it’ll be a Nobel Prize and that’s enough for me’.
I certainly wish her luck.