Physicists and Astronomers have had a big problem now for a very long time. Once astronomer Carl Hubble recognized that a large number of the fuzzy objects out in space called nebula were in fact entire galaxies astronomers and astrophysicists starting trying to work out the dynamics of how those galaxies behaved. Take a typical spiral galaxy like our own Milky Way, it has a central globe about 20,000 light years in diameter surrounded by a thin disk 200,000 light years in diameter but only about 5,000 light years thick, see image below. The density of stars is greatest in that central sphere and slowly but steadily decreases the further out you go along the disk.
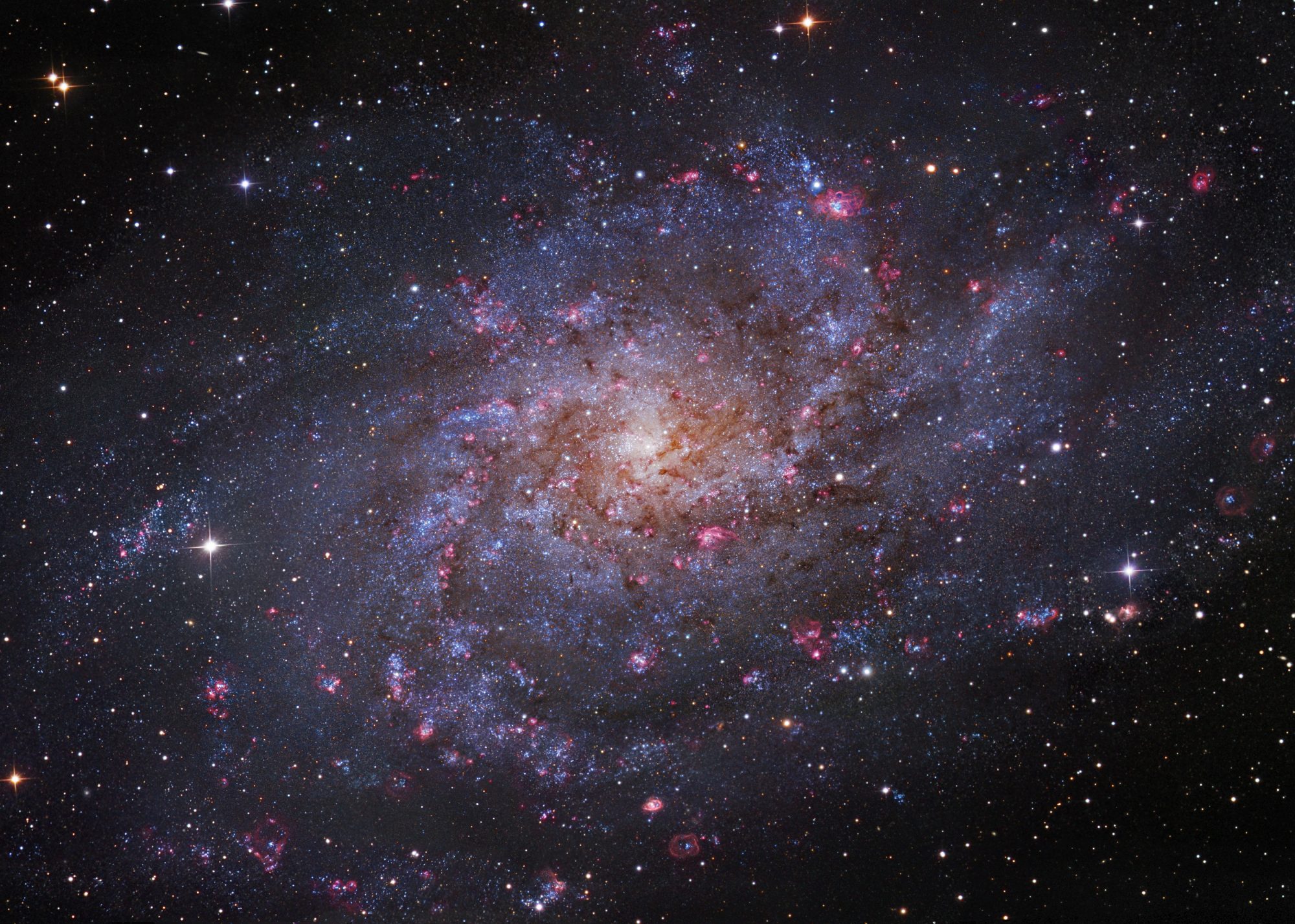
Physicists immediately recognized that such a galaxy would only be stable if all of its stars orbited around the center and indeed our Sun is calculated to orbit around the Milky Way’s center once every 200 million years. If astronomers could estimate the mass distribution of the stars then the physicists could use Newton’s law of gravity to work out a velocity profile for the galaxy. Basically that would give them a formula for the velocity a star would have as a function of its distance from the center of its galaxy. That formula could then be checked by using the Doppler effect to measure the actual velocities of stars at various places in a galaxy.

It didn’t work. Dozens of studies, dating back to 1933 have shown that the stars near the outer edge of a galaxy are moving too fast. Indeed the whole profile of velocity versus distance indicated that galaxies should have more than twice the mass that we can see and that mass should be spread out more evenly from the center.
That’s where the idea of ‘Dark Matter’ comes from, some form of matter that doesn’t radiate light but possesses a great deal of mass. There have been a lot of ideas about what dark matter could be, several of which have even been given ‘cutesy’ names. MACHOS, which stands for Mass Concentrations, could be anything from brown dwarfs, objects too small to become stars but larger than planets, to stellar mass black holes. Problem with either alternative is that you need huge numbers of them; remember we have to more than double the mass of the galaxies. Astronomers have found a few brown dwarfs and stellar mass black holes but nowhere near enough to solve the problem of the missing mass.

Then there are the WIMPS or Weakly Interacting Massive Particles. These are elementary particles like electrons or quarks except that they are electrically neutral so they don’t interact with light, and they are very massive. Particle physicists like WIMPS because they can connect them to the particles predicted by theories of ‘Super-Symmetry’. Problem is that despite decades of searching, and building powerful particle accelerators like the Large Hadron Collider (LHC) at CERN no evidence for any super-symmetric particles has ever been found.
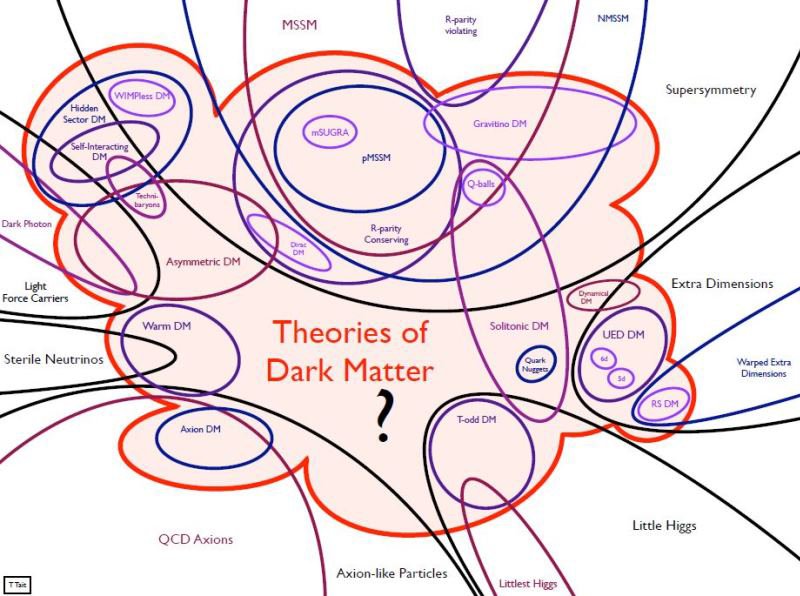
A completely different approach was taken back in 1983 by Israeli physicist Mordehai Milgrom. Maybe he asked, Newton and Einstein were wrong about gravity, and then Milgrom proceeded to modify the gravitational field equations so that they would accurately predict the behavior of galaxies. Milgrom referred to his theory as MOdified Newtonian Dynamics or MOND the name by which it has since been known. If MOND or some similar altered theory of gravity is true then the failure to detect dark matter is easy to understand, there simply is no such thing as dark matter, gravity is different than what we thought.

Now according to Newton and Einstein gravity obeys what is known as an inverse square law, see equation. 1. This means that the strength of gravity gets weaker the further two masses are by the square of the distance between them. Double the distance and gravity is one quarter as strong, triple the distance and gravity is one ninth as strong, four times the distance yields one sixteenth the strength and so on.
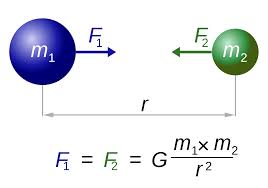
The changes Milgrom proposed to the inverse square law where very small. They had to be because Newton-Einstein works extremely well in our solar system and recently astronomers have even shown that stars orbiting around the supermassive black hole at the center of our galaxy follow Newton-Einstein very accurately, see my post of 6May2020. Crucially however, the small change proposed by Milgrom doesn’t grow weak as quickly with distance as inverse square. This means that at enormous distances, much larger than our solar system, tens to hundreds of light years, it is the modified term that starts to dominate over the Newtonian inverse square term.
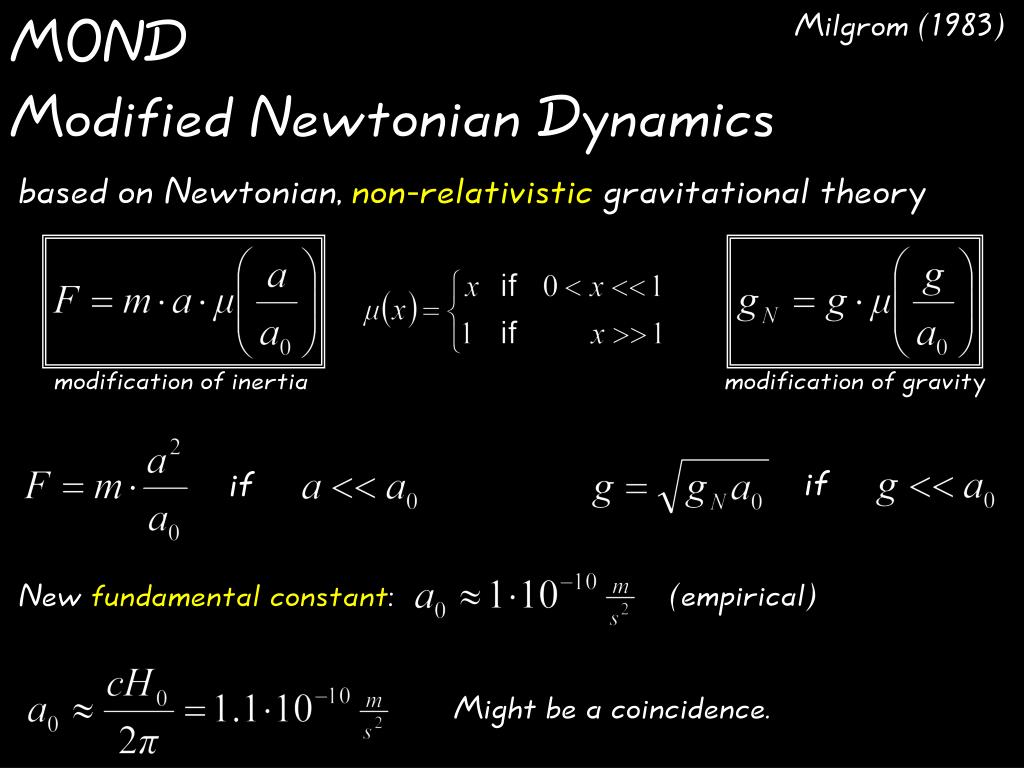
All that makes it very difficult to test MOND or any similar small changes to Newton-Einstein. There is one difference however that just might be measured. One of the quirks of a pure inverse square law is that if you are sitting at the center of a mass distribution then you are being pulled by the gravity of those masses equally in every direction so that you literally feel no force! Think about it, if you are at the center of a planet there is a lot of matter all around you but you’re being pulled down just as much as up, back just as much as forward and to the left just as much as to the right. Being pulled equally in every direction you end up not being pulled in any direction, so you feel no gravity. This is known as the lack of effect from an external field.
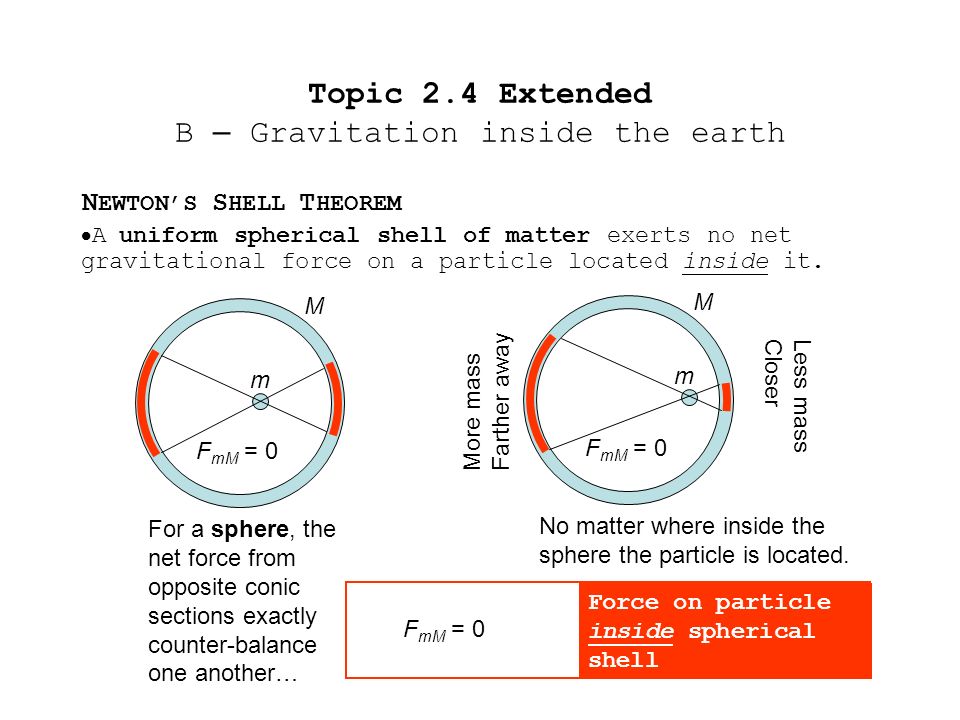
In MOND however an external field can be felt and so the rotation curve of a galaxy at the center of a large cluster of galaxies would differ from the rotation curve of a similar galaxy that is far from any other large galaxy. To test this idea a group of astrophysicists from Sejong University in South Korea, Cardiff University and the University of Oxford in the UK along with Chase Western Reserve University and the University of Oregon in the USA has examined the rotation curves of 153 galaxies to see if there is any trace of such a difference. The study is entitled ‘Testing the Strong Equivalence Principle: Detection of the External Field Effect in Rotationally Supported Galaxies’ and has been published in the Astrophysics Journal.
What they discovered was that of rotational speeds of galaxies inside an external gravitational field were slowed when compared to the rotations in more isolated galaxies, something contrary to Newton-Einstein but exactly as predicted by MOND. Statistically the results so far give a 4σ confidence level, just below the golden 5σ confidence that physicists use to declare a ‘discovery’. With results so provocative you can be certain that the researchers will be working to both find more evidence as well as improve the data they already have.
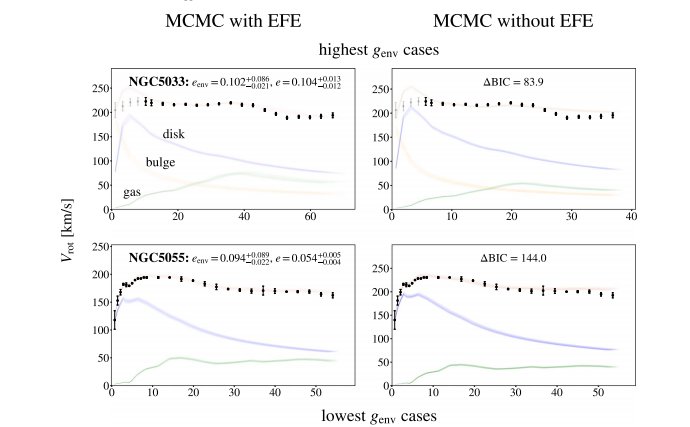
If MOND does turn out to be correct it will not only eliminate the need for dark matter it will force a reevaluation of many other well established theories. Much of Cosmology and the Big Bang Theory are rooted in Einstein’s gravitational field equations but so far no one has ever been able to expand MOND to describe the Universe as a whole. So even while MOND has gained strong new evidence in its favour there’s still a long way to go before it becomes generally accepted by the majority of physicists. The problem of galactic rotation has been around a long time and it looks like it will continue to be so for a little while longer.