A freshman physics class in any college or university will for the most part deal with the movement and behavior of particles, that is objects with easily measurable and long lasting quantities like size, shape, mass, position etc, etc. As someone who grew up in a baseball family I freely admit that whenever I encounter the word ‘particle’ the first thought in my brain is something very like a baseball.
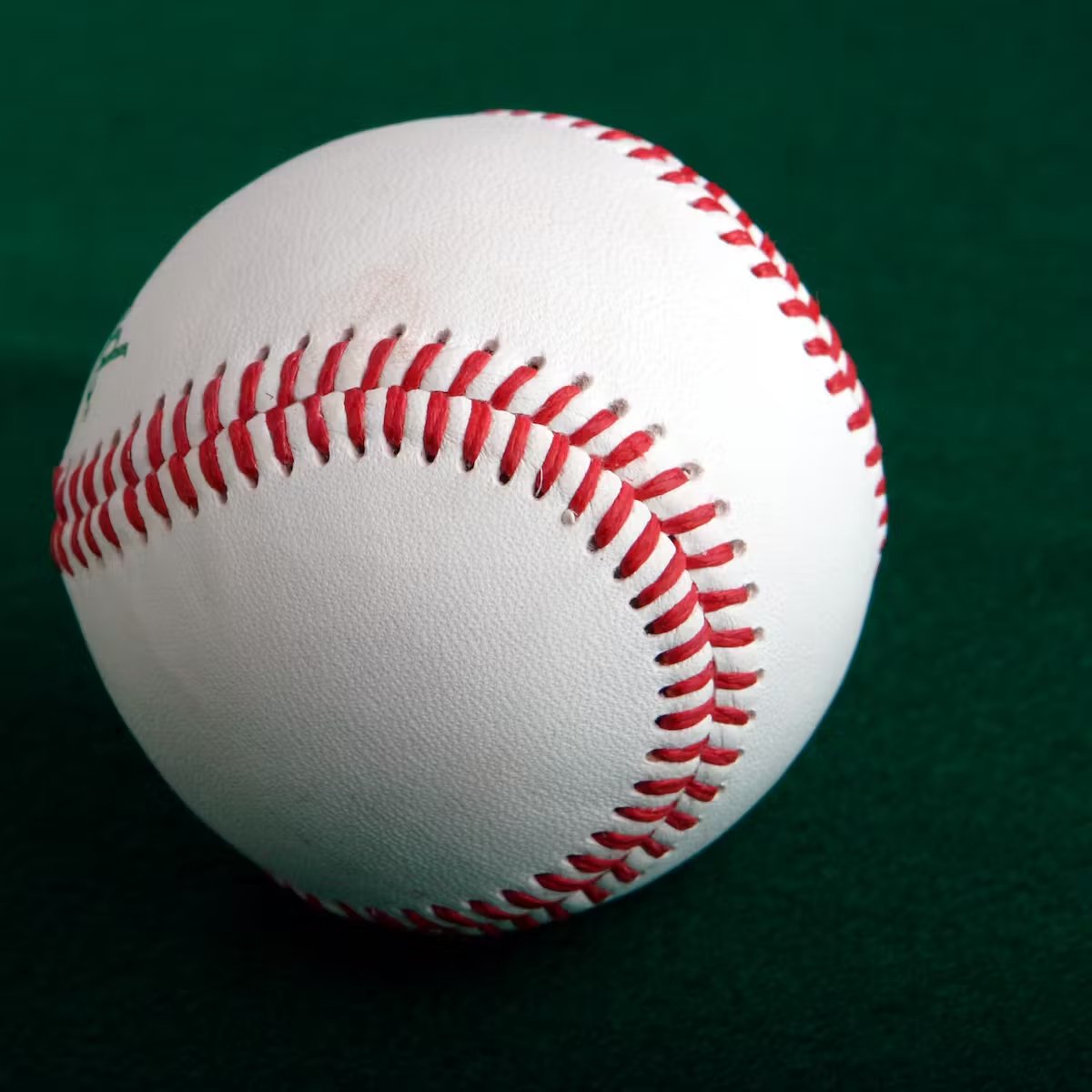
In that freshman physics class the students will learn about such other particle quantities as velocity, acceleration, and momentum while discussing collisions between particles, the forces between particles and so on. In other words freshman physics talks a lot about particles.
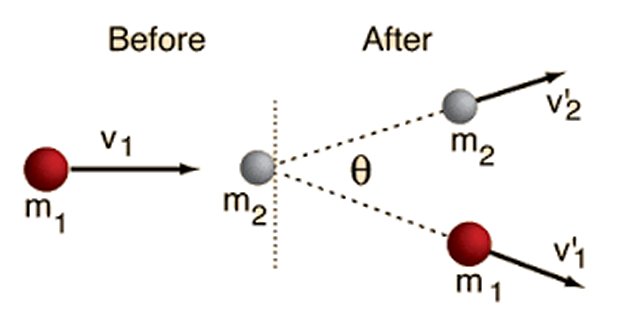
Higher level physics is pretty much the same. Experimental physics is usually conducted at High Energy Particle Accelerators like the Large Hadron Collider at CERN (A Hadron is a kind of sub-atomic particle) or deep underground where ‘Ghost Particles’ called Neutrinos are captured in huge vats of water. Today I’ll be discussing some recent studies in particle physics, the first concerning those ghost particles the neutrinos while the second concerns objects you might consider it strange to call particles, human beings.

Neutrinos have fascinated physicists ever since Wolfgang Pauli first predicted their existence in order to ‘balance the books’ in beta radiation decay. Originally thought to be a single kind of particle with no electric charge and either no or very little rest mass (Neutrino = little neutral one in Italian) they have only gotten stranger as we’ve learned more about them. We now know that there are at least three distinct types, or generations of neutrino, the electron, muon and tau each named for the type of electron like particle they are generated with. Unlike other generations however, up, charm and top quarks for example, the three types of neutrino can oscillate from one type into the other into the other. This implies that neutrinos must have some rest mass, perhaps one millionth that of an electron.

Like other Fermions each type of neutrino also has an anti-particle, or does it? You see in general anti-particles have the exact same mass but the opposite electric charge of their species of particle. However, since neutrinos have no electric charge there is a real possibility that neutrinos may be their own anti-particle and that would certainly be new physics.
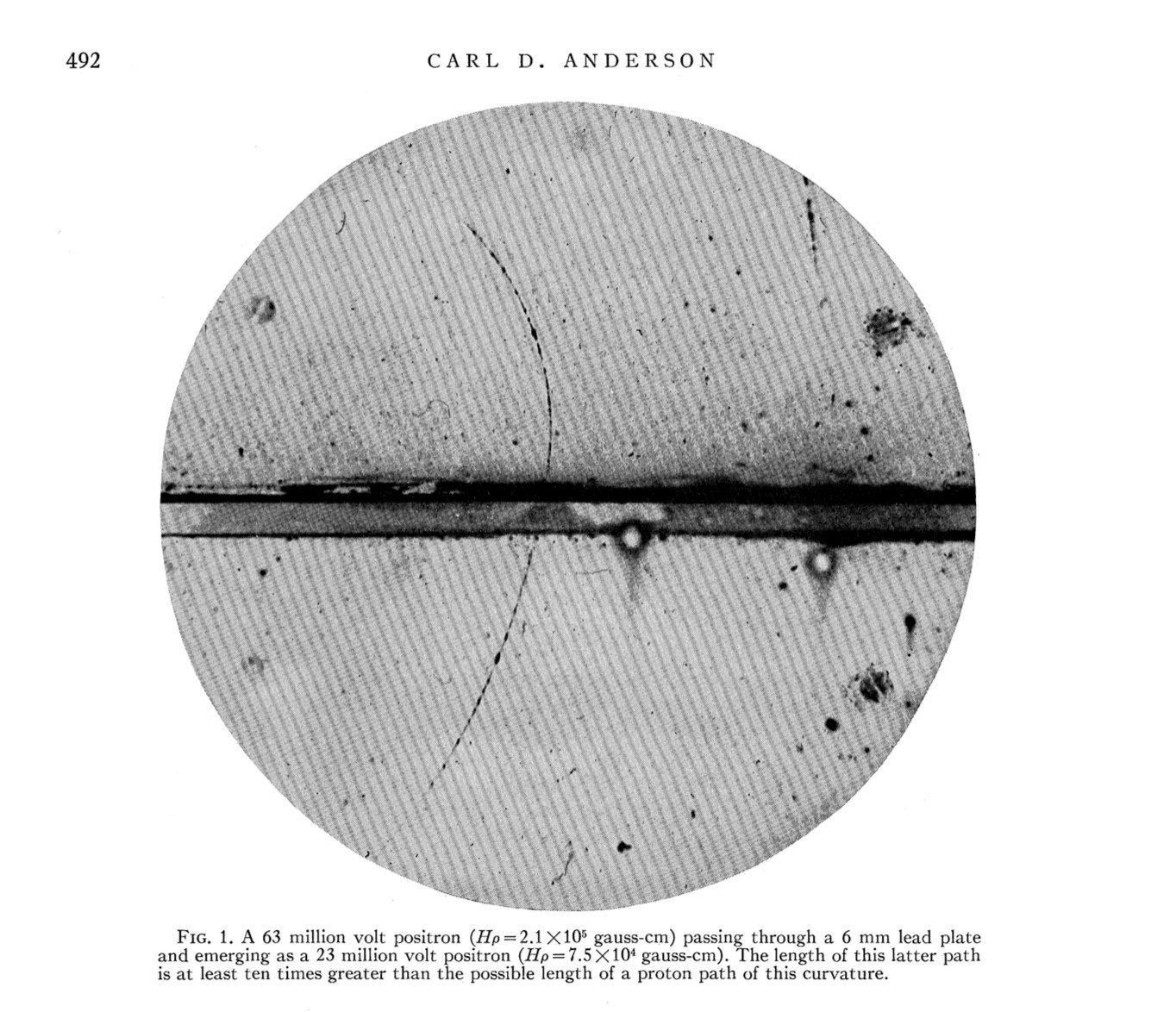
Now neutrinos are very difficult to study, they only rarely, very rarely interact with other kinds of particles. It’s estimated that the neutrinos generated in the Sun by fusion could go through a light year of solid lead and half would still come out the other side. Physicists who study neutrinos usually need sources of trillions of the particles in order to catch just a few.
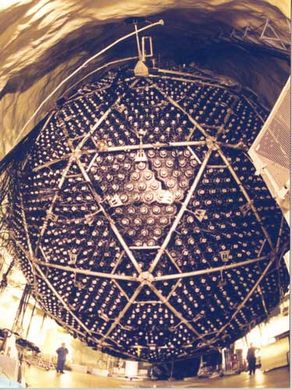
Experimentalists decided that their best chance for determining if neutrinos were their own anti-particle was to use a very rare type of radioactive decay called a double beta decay that occurs in the isotope 76 of the element germanium, atomic number 32. Double beta decay happens when the nucleus emits two electrons and two anti-neutrinos at the same time while jumping up two spots in the periodic table to selenium, atomic number 34.
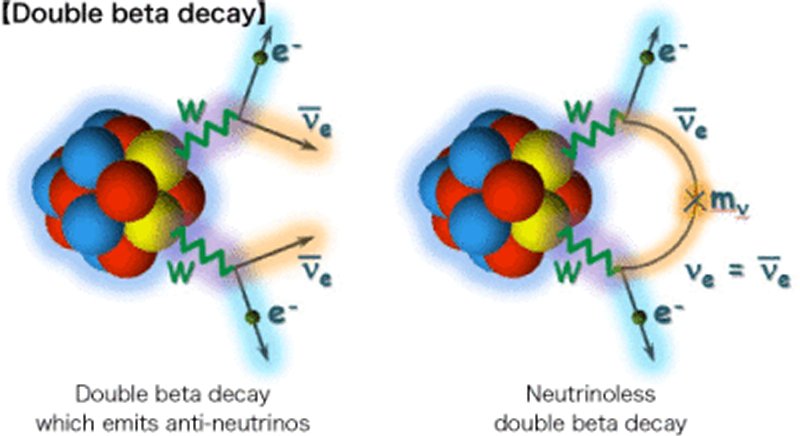
If the neutrino is its own antiparticle however then the two anti-neutrinos could annihilate each other and only two electrons would come out, electrons, the easiest particle to detect and measure. So what the experimentalists would have to do is measure the mass / energy of the germanium nucleus before the decay, and the mass / energy of the selenium nucleus after the decay and if the two electrons got all of the energy difference then there were no neutrinos and the neutrino is its own anti-particle.
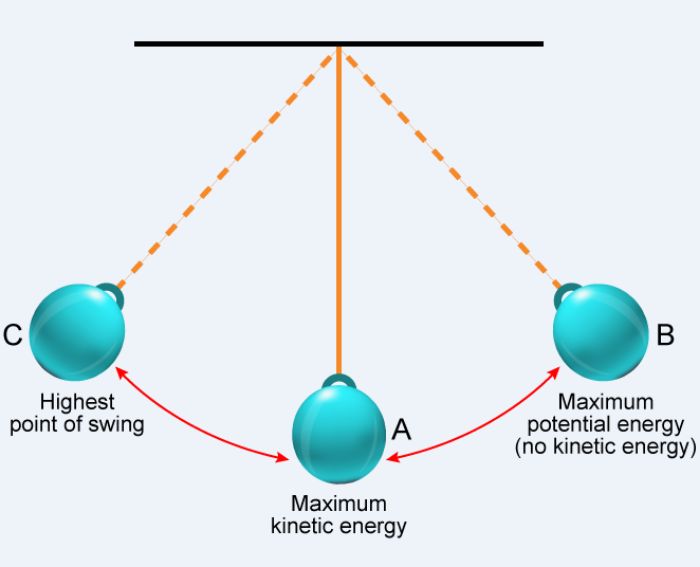
Easier said than done. The experiment to search for neutrinoless double beta decay (0νββ) was carried out using 30 kg of germanium that had been enriched to 88% isotope 76, in nature isotope 76 makes up 7.75% of all germanium. The germanium was then surrounded by detectors to both find and measure the energy of any electrons that were emitted. In order to reduce as much as possible interference from other radioactive decays the experiment was conducted a kilometer and a half beneath the Earth’s surface at the Sanford Underground Research Facility in South Dakota, an old abandoned gold mine. After ten years of operation the collaboration of scientists that runs the experiment has just released its results, no neutrinoless double beta decays were observed by the experiment which sets new limits on the possibility that a neutrino could be its own anti-particle.
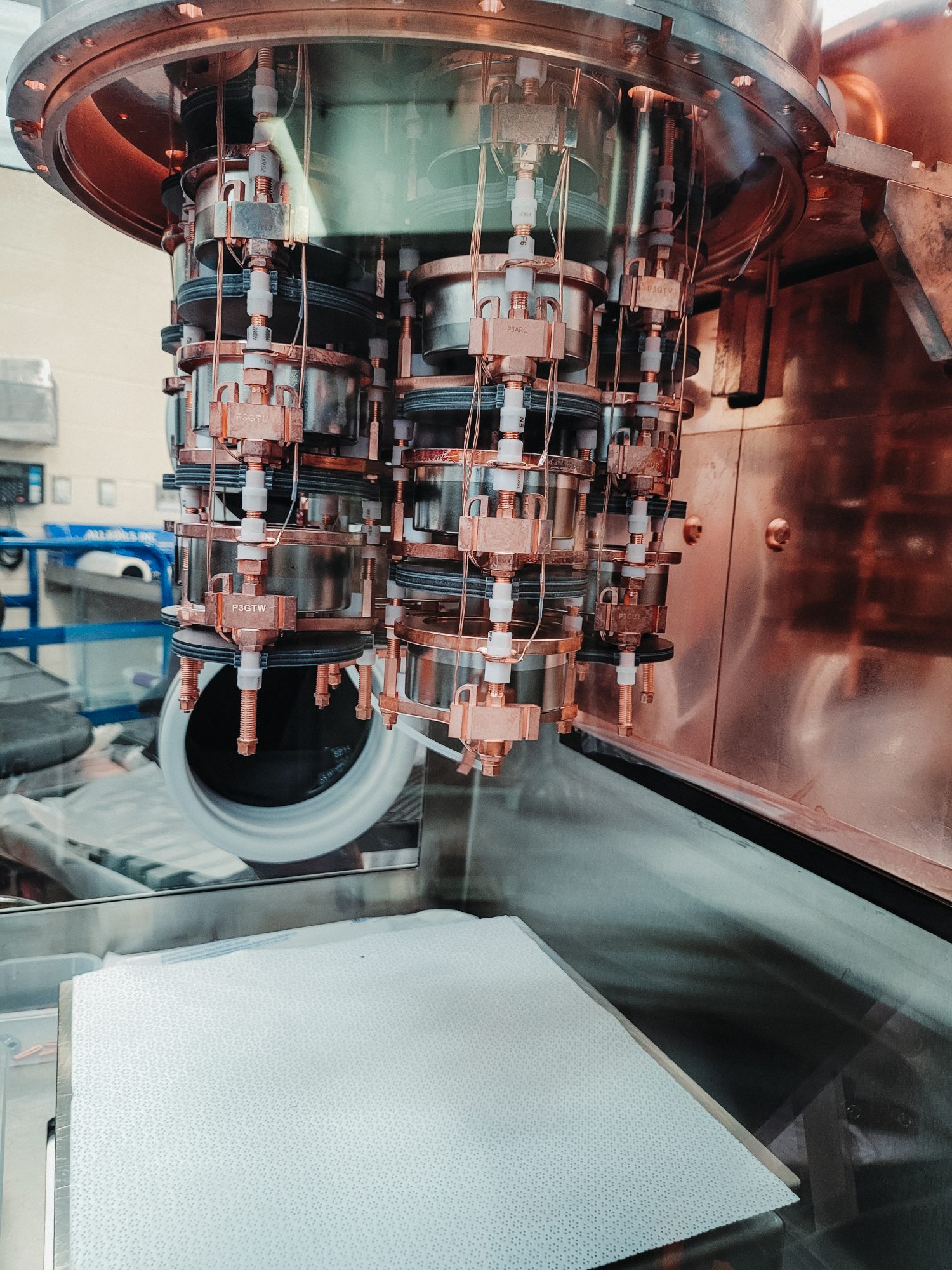
Of course just because you haven’t found something doesn’t mean that it doesn’t exist, it could mean you just haven’t looked hard enough. So the physicists who ran the experiment are now planning a new experiment that will employ 1,000 kg of germanium in their search to learn more about the ‘Ghost Particle’.

Human beings are not generally thought of as ‘particles’ but in many ways can be treated as such. After all we each have a definite size and shape as well as mass so there are many circumstances where the involuntary motion of a person is exactly like any particle. That’s why we can use ‘crash test dummies’ as substitutes for real people in automobile safety testing because during the conditions of a crash the humans inside the car are really just particles.
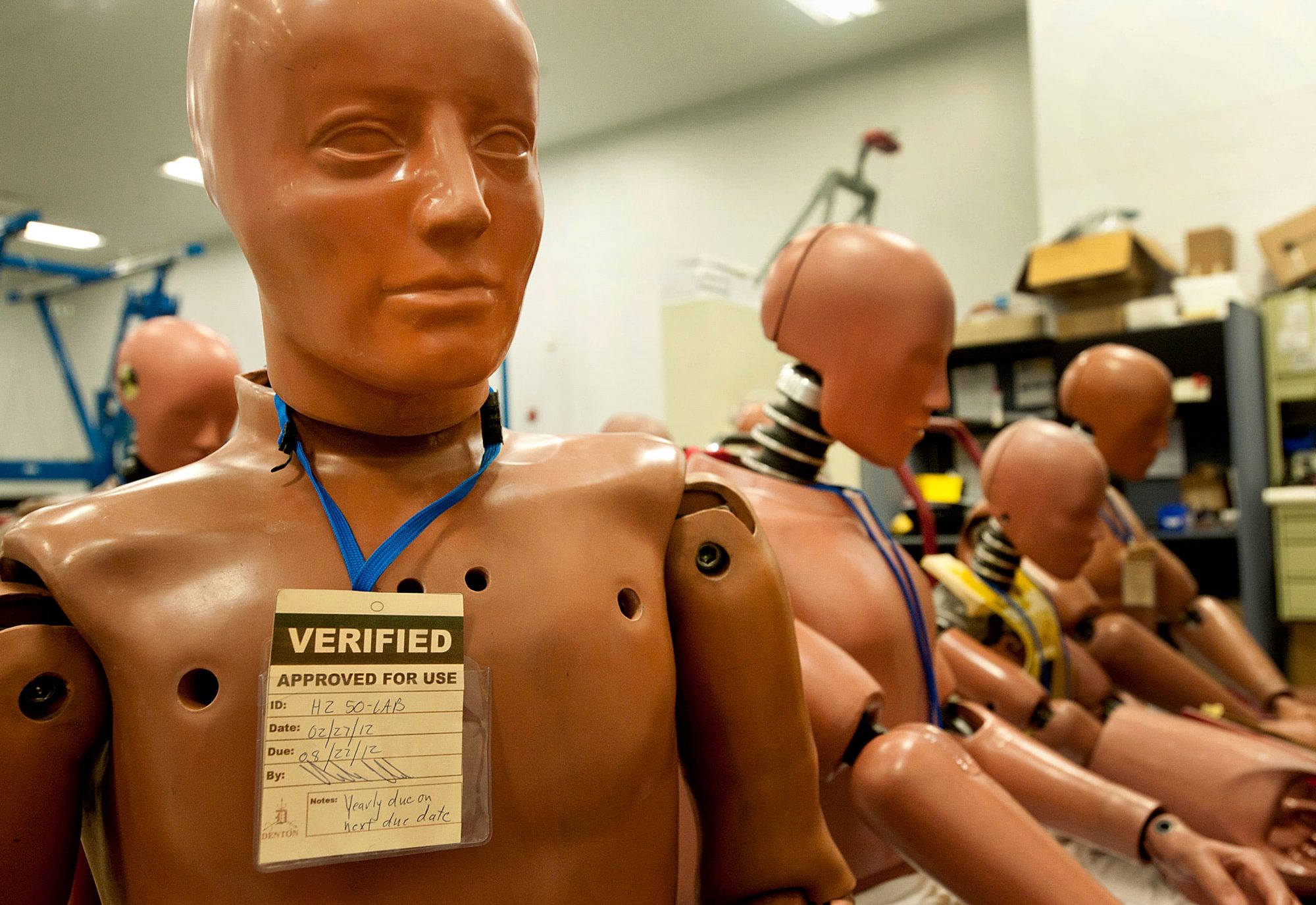
There are even times when the voluntary motions of humans can be studied as particles and something of the laws governing that behavior learned. One well known example of this is when two groups of people, traveling in opposite directions have to move through each other, such as when two groups of pedestrians are trying to cross to opposite sides of the same street using the same crosswalk and have to go through each other.
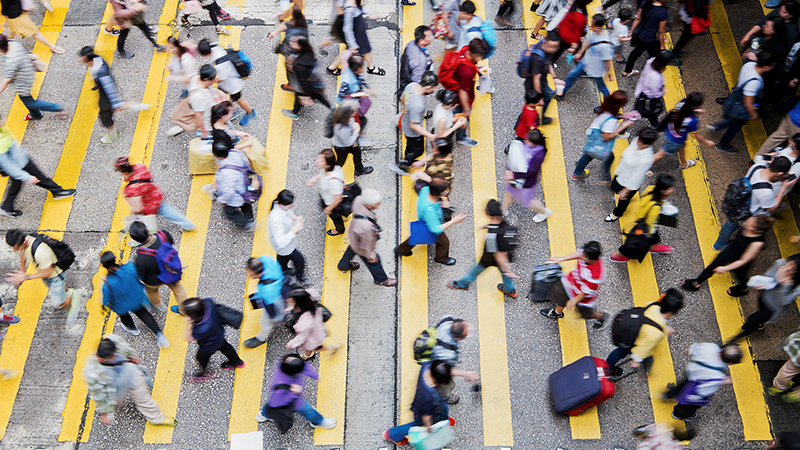
What has been observed in such circumstances is that the two groups will break up into a series of ‘lanes’ that will interleave with the lanes of the opposing group. These lanes then move past each other in what is technically known as two component flow. This type of phenomenon in general has been given the name ‘active matter’ and has been observed in many different kinds of animals from flocks of birds to schools of fish.

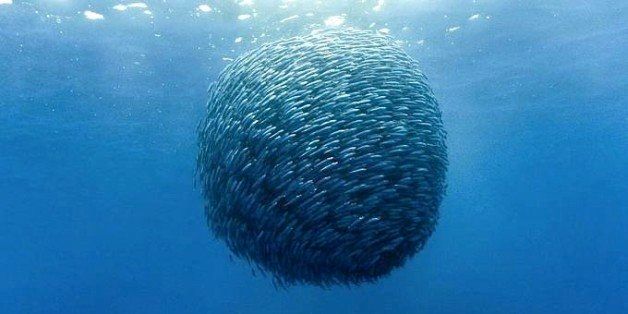
While the development of lanes in groups of people moving in opposite directions has been studied for several decades no rigorous mathematical model of the behavior had been published. Until now, for a new paper in the journal Science by mathematicians at the University of Bath in the UK has presented a kinetic description of lane nucleation, in other words equations describing how lanes form and behave. The model was in fact based upon Albert Einstein’s description of ‘Brownian Motion’ of pollen grains in a solution.
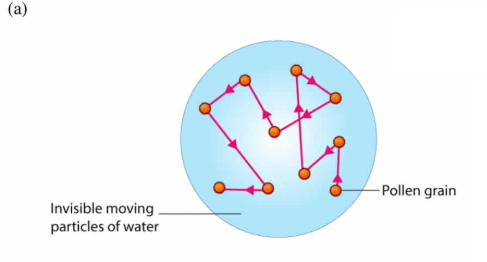
The mathematicians have even teamed up with experimentalists at the Department of Human Motor Behavior at the Academy of Physical Education in Katowice in Poland to test their model. One of the experiments was set up in King’s Cross Station in London where groups of volunteers moved through different gates and obstacles. The movement of the volunteers was video recorded and in every case order arose out of chaos allowing the recordings to be compared to the model’s predictions.
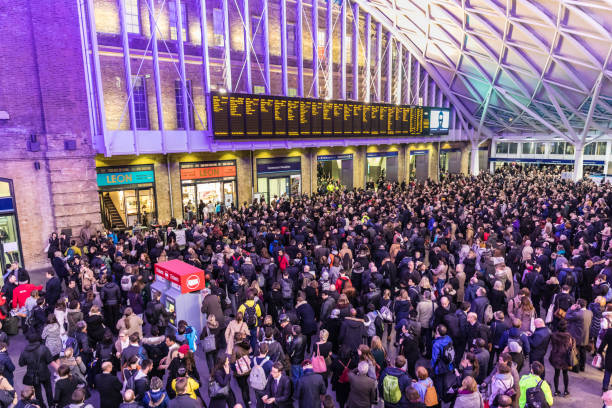
So it seems that the idea of a particle, so useful in physics, can also be applied to the study of living creatures, even we humans.