Everyone has heard a little bit about Supernovas, you know those stars who destroy themselves in explosions that for a few weeks can outshine many billions of normal stars. Supernovas are very rare events, happening only every couple hundred years in our galaxy of one hundred billion stars. In fact supernovas are so rare that most of what we’ve learned about them comes from observing ones that happen in other galaxies. It works like this, if supernova only occur once every 100 years per big galaxy then if you keep an eye on 1,000 galaxies you should see about 10 a year!

The very first studies of supernovas, conducted more than 70 years ago now, used spectral analysis to show that there were two basic types. One type, not surprisingly called Type 1, had virtually no hydrogen in the spectra obtained from their light. Now hydrogen is the most common element in the Universe so for Type 1 supernovas to be completely lacking in it is really significant. Type 2 supernovas are just the opposite, their spectra shows plenty of hydrogen. One thing both types have in common is that they are very rare which indicates that only a small percentage of stars ever go supernova.
In time astrophysicists came up with two rather different models of supernovas. Type 1 begin with a white dwarf, the superdense corpse of a once normal star, for example our Sun will become a white dwarf in about 6-7 billion years when it runs out of its hydrogen fuel. A typical white dwarf has a mass about that of our Sun but its size is only that of the Earth. The surfaces of dwarfs are extremely hot but because of their small size they are much dimmer than a normal star like our Sun.
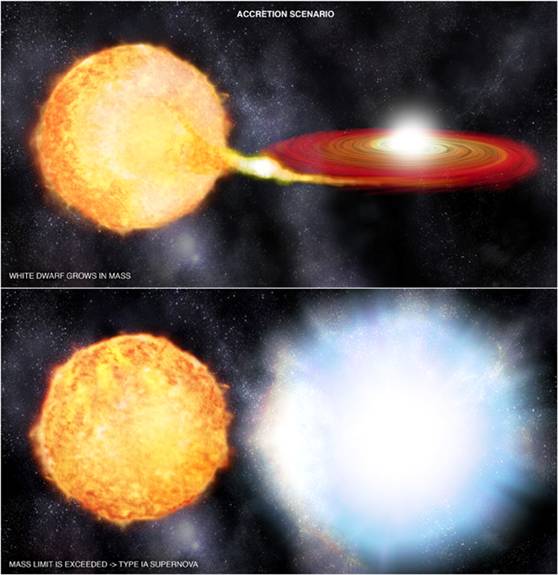
Now if a white dwarf happens to have a companion star, and there are many examples of binary star systems, the dwarf can start pulling material away from its companion. This stealing of matter can only go on so long however because there is a maximum limit to the mass of a white dwarf. This maximum mass is about 1.4 times the mass of our Sun and if a dwarf exceeds this limit it begins to collapse triggering the Type 1 supernova. After the explosion all that’s left of the star is a neutron star or even a black hole.

Type 2 supernova however start as huge, very massive stars, at least ten times the mass of the Sun. The fusion reactions in such stars use up their hydrogen fuel in only a few million years. The star will then begin to fuse helium into carbon and oxygen, which is as far as our Sun will ever get. Massive stars however have enough energy to keep going, fusing carbon and oxygen into heavier elements all the way up to iron.
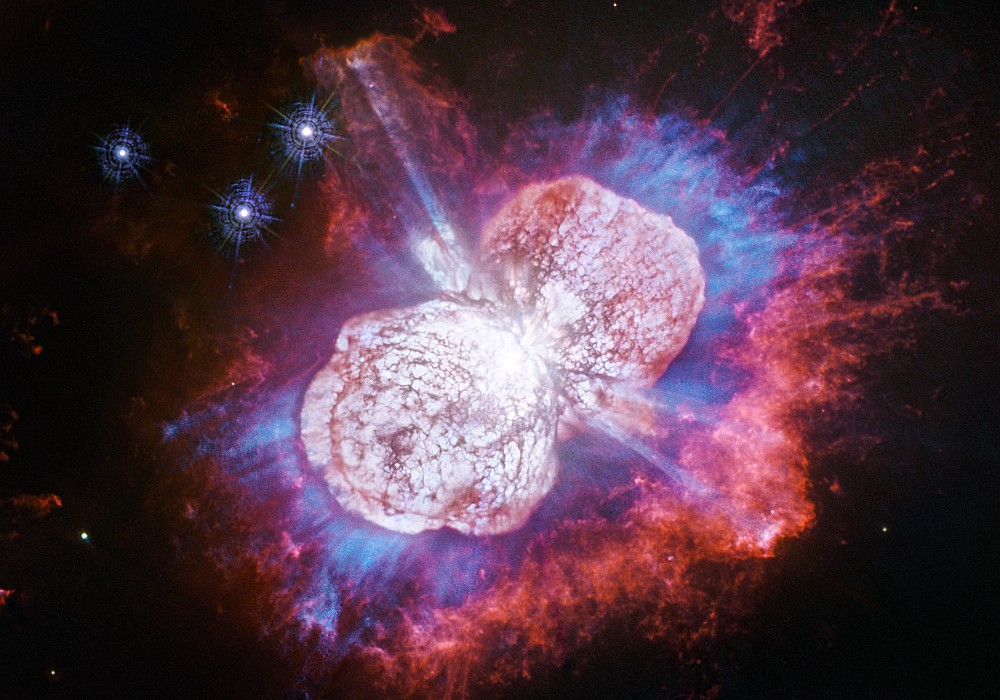
Iron is a brick wall however, fusing iron into a heavier element doesn’t produce energy it consumes it. The fusion reactor of this huge, massive, intensely hot star suddenly comes to a screeching halt and the star begins to collapse upon itself. This collapse triggers the supernova but unlike a Type 1 supernova there is still some hydrogen left in the star’s outermost regions, which shows up in the explosion’s spectra. After the explosion all that remains of the star is a neutron star or black hole.

Those are the theories, but to be certain they’re right we would have to observe a star before it goes supernova and that’s not an easy thing to do. After all there are literally trillions of stars in our galaxy and nearby ones, while only a couple of dozen of those stars will go supernova each year. The question is then, which ones? Well what astronomers have tried to do is to get observations of as many stars as possible. Then when a supernova does occur they check their archives to see if they have any prior images of it.
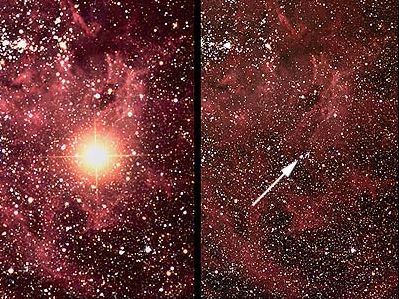
The first time that this technique worked was the Type 2 Supernova SN 1987A, in the Large Magellanic Cloud, which is a satellite galaxy orbiting the Milky Way. Almost as soon as SN 1987A was detected astronomers quickly began looking through their past observations and succeeded in finding a few observations of the star, catalog name SK-69º202 before it exploded. Although there were a few surprises SK-69º202 turned out to be pretty much what astronomers had expected, with 15 times the mass of our Sun and a very hot surface. The data gained from SN1987A taught astrophysicists a great deal about Type 2 supernovas, but of course they wanted more, and in particular, they wanted a Type 1 supernova progenitor.
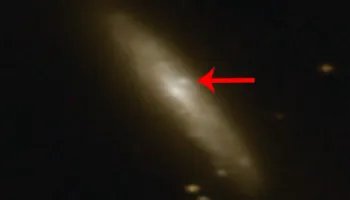
Now they may have one, and it’s not what they expected. Back in December of 2019 astronomers spotted a supernova, designated SN 2019yvr in the galaxy NGC 4666 which is about 46 million light years away in the Virgo super cluster of galaxies. Even as observations were showing that SN 2019yvr was a Type 1 supernova astronomers associated with the Hubble Space Telescope were rummaging through earlier images of NGC 4666 to try to see if Hubble had ever made any observations of the star.

The astronomers spent more than a year of checking and crosschecking between the measurements made after the supernova began to those that had been taken earlier. Nevertheless they think they may have found the supernova’s progenitor in a series of images taken some 2.6 years before the explosion, problem is, the star they’ve identified is not the kind the theory says it should be.
Instead of a tiny, dense, extremely hot white dwarf the star that’s been identified is a fairly cool orange-yellow star more than 300 times the width of our Sun. A star like that should have plenty of hydrogen left in it but the spectra of the supernova showed none, it’s just a mystery.
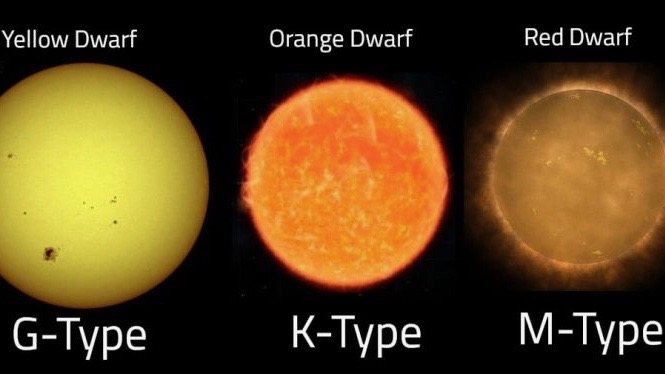
The astronomers have already come up with several explanations for the disagreement with the theory. First of all they could simply have identified the wrong star. They can’t check to make certain right now because the debris of the supernova is currently obscuring that region of space and it will take 5-10 years before they are able to see if the orange-yellow star is still there.
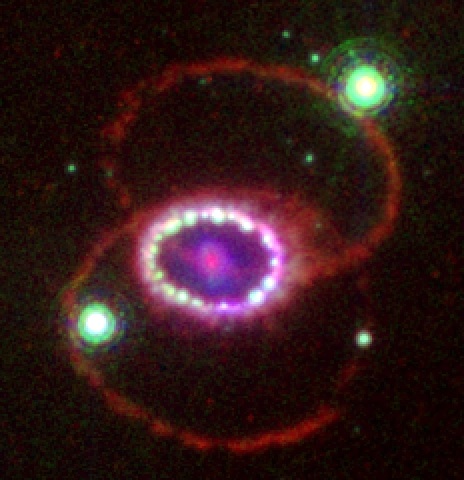
Then there’s also the possibility that the companion star, remember Type 1 supernovas require a companion star, could have given off enough material to form a cool gaseous shroud around a white dwarf that was the actual supernova progenitor. Again checking this possibility will have to wait for the debris to clear a good deal.
Of course there’s also the possibility that our theories are just wrong and have to be adjusted. Whichever possibility turns out to be true astronomers are bit by bit learning the secrets of what are some of the most spectacular events in the Universe, Supernovas.