Let me begin today but reminding everyone of the problem of Dark Matter. Over the last 70-80 years as astronomers studied the dynamic behavior of the galaxies they found that the gravity of the objects that they could see, i.e. the stars that shined, was not sufficient to account for the way galaxies moved. There had to be some form of missing mass, some kind of dark matter in galaxies in order to explain their dynamics.
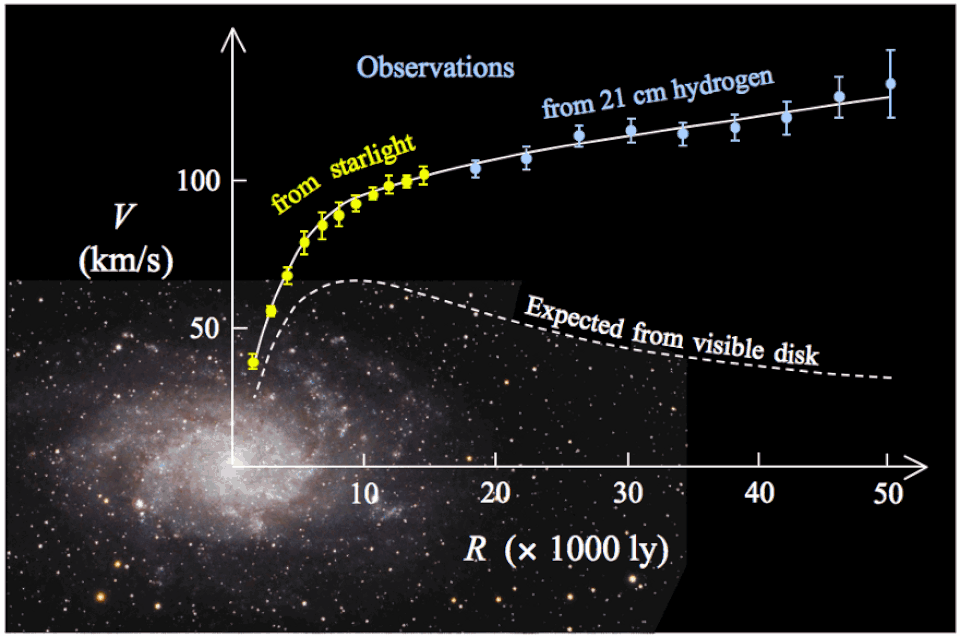
Back in the 1980s when I was an undergraduate the ideas about Dark Matter had basically coalesced into two types of matter. These two classes of matter were given the corny names of Machos, meaning Mass Concentrations, and WIMPS meaning Weakly Interacting Massive Particles. Mass Concentrations were thought to be composed of ordinary particles like protons, neutrons and electrons and could be anything from small black holes to dark stars, given the name brown dwarfs, or even smaller objects like planets.

Now astronomers didn’t like the idea of having to look between the stars for small objects that didn’t shine by their own light, so they didn’t like Machos. Let’s face it telescopes are the main tool of astronomers and telescopes gather light from objects that shine like stars.
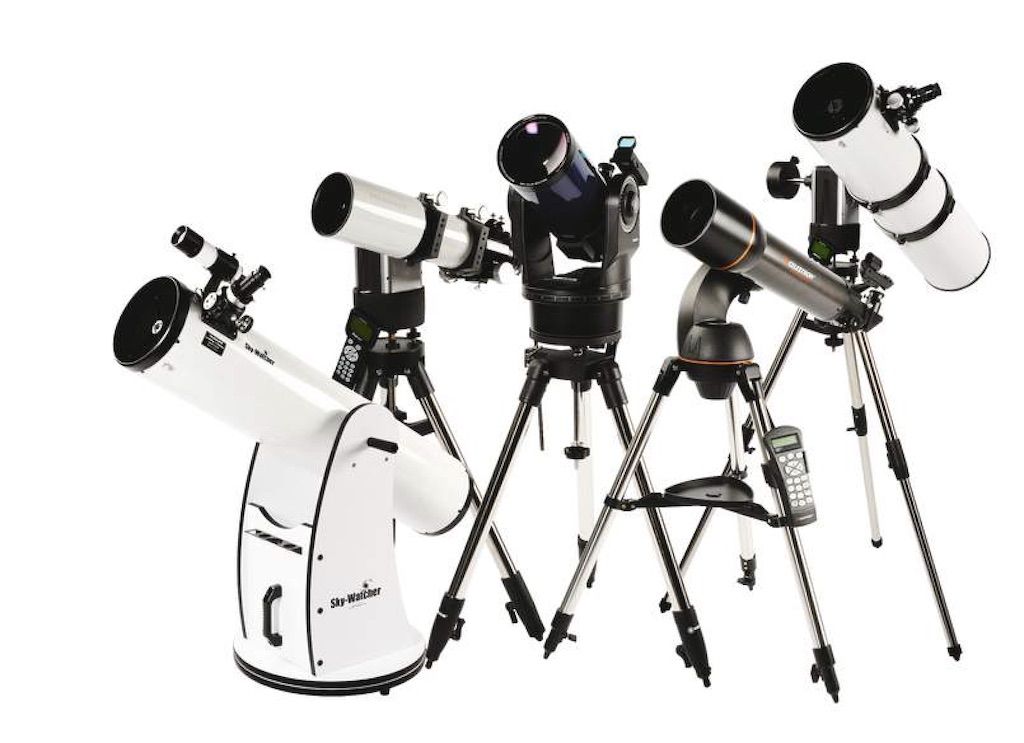
On the other hand physicists loved the idea of WIMPs because at the time they were coming up theories of ‘Supersymmetry’ that predicted the existence of a large number of massive particles some of which could be WIMPs. So starting in the 1990s Machos were largely ignored while everybody went looking for WIMPs either in outer space or at the big atom smashers like the Tevatron at Fermilab or the new Large Hadron Collider at CERN.
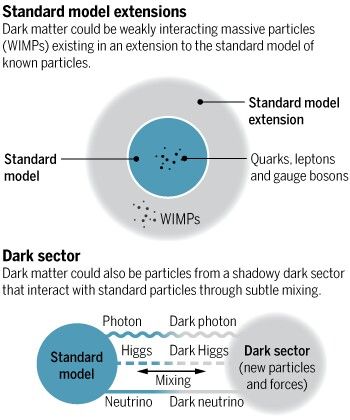
Problem is that after thirty years of searching no particles that could be WIMPs have been found. And now it seems that the wind has shifted and maybe it’s time to take another look at Machos.
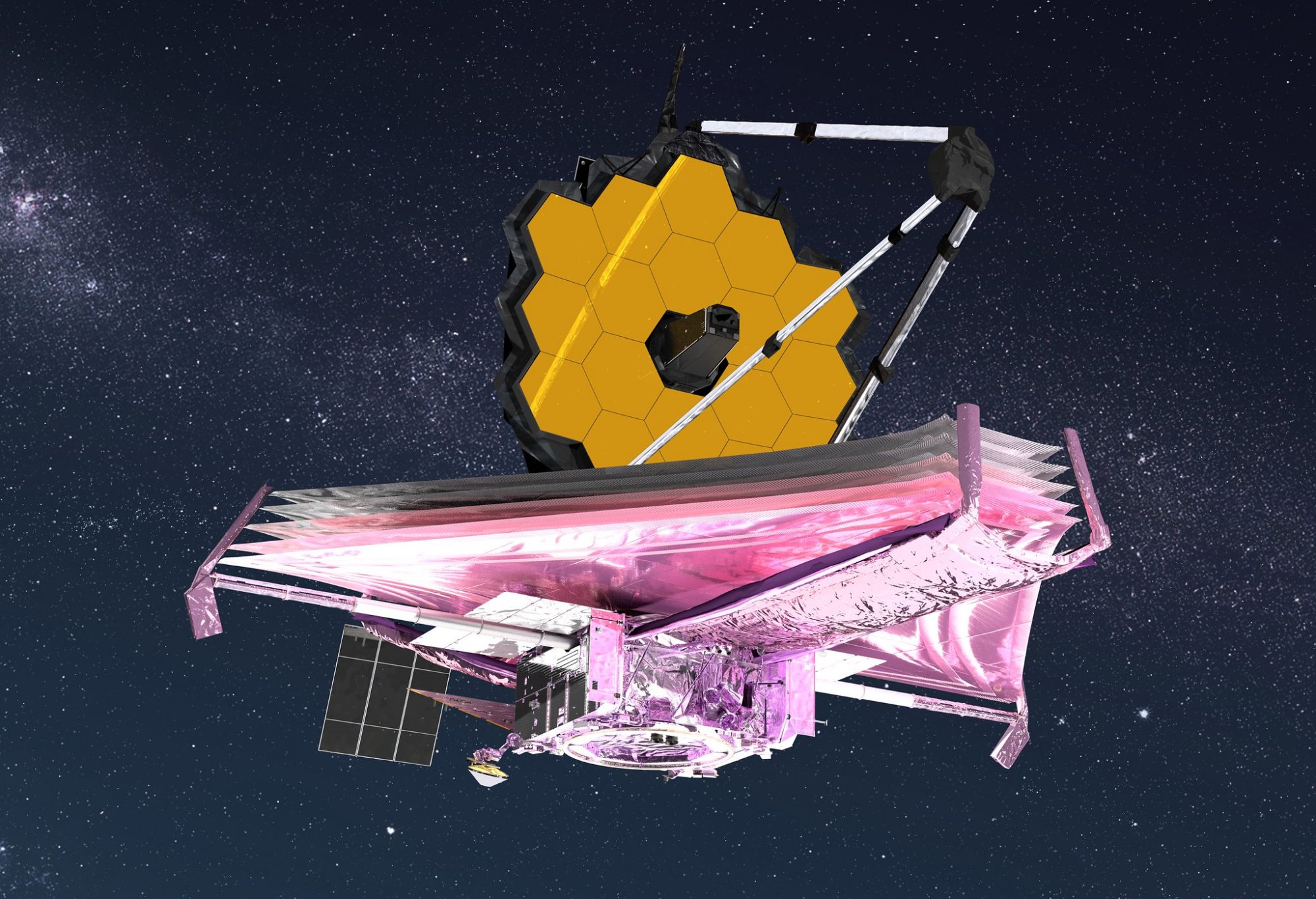
For one thing astronomers have new, bigger, better instruments that are more capable of looking for objects that don’t shine at visible wavelengths. Just a few months ago I published a post about how astronomers are beginning to discover large numbers of Brown Dwarf stars, objects too big to be called planets but too small to ignite nuclear fusion in their cores so they do not shine like a star. See my post of 22 September 2021.

Now a new study from the European Southern Observatory in Chile and Bordeaux University has announced the discovery of as many as 170 rouge planets, that is planets that do not orbit any star but rather move through the Milky Way all on their own. The rogue planets were discovered in a star forming region of the galaxy relativity close to our solar system in the constellations of Scorpius and Ophiuchus.

It was the fact that the rogue planets were very young, and therefore still warm that enabled the astronomers to find them in the infrared region of the electromagnetic spectrum. Even so the astronomers at Bordeaux had to shift through observations accumulated over 20 years and still aren’t certain how many rogue planets they’ve found, the best estimate being 70 to 170 Jupiter sized worlds.
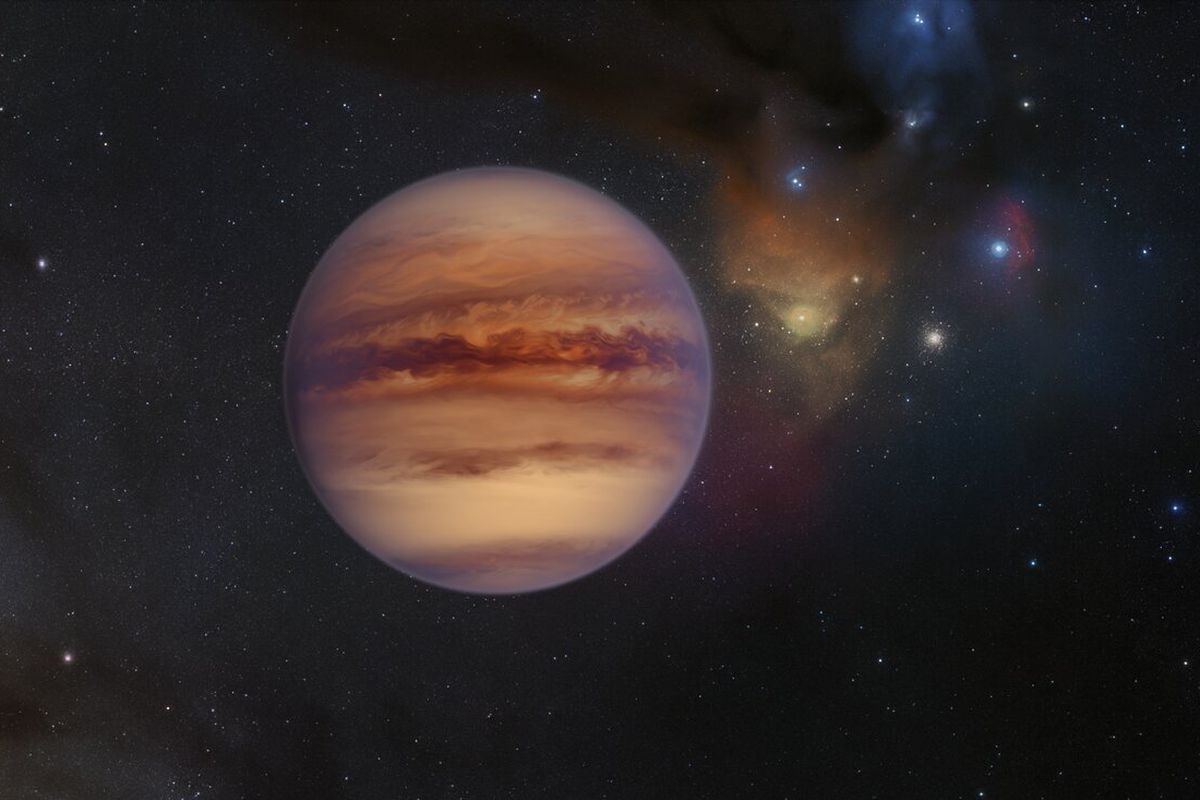
Still if the star forming regions in space are also producing large numbers of solitary planets who knows how many older rogue planets there could be between the stars. Could there be as many rogue planets as there are stars? Or maybe even more? Finding out just how many rogue planets there really are could be a difficult task, remember once the planet cools down like our earth did after a few million years they’ll be almost impossible to find.

And there’s one more candidate for a Macho because the possibility that there could be large numbers of small, ‘primordial’ black holes in the Universe is once again being seriously discussed. These would be black holes with a mass that of a planet that formed in the first seconds after the big bang and have just been floating around ever since then. Such black holes would also be very difficult to find, unless of course one of them came inside our solar system.

So maybe we don’t need physics beyond the standard model in order to explain Dark Matter. If there are a lot more Brown Dwarfs than we ever imagined, more Rogue Planets and more primordial Black Holes maybe Dark Matter is just protons, neutrons and electrons in objects that don’t shine by their own light.
Machos may not be as exciting as WIMPs, but reality is what it is and after thirty years of failing to find any exotic elementary particles maybe we need to give Machos a rethink!